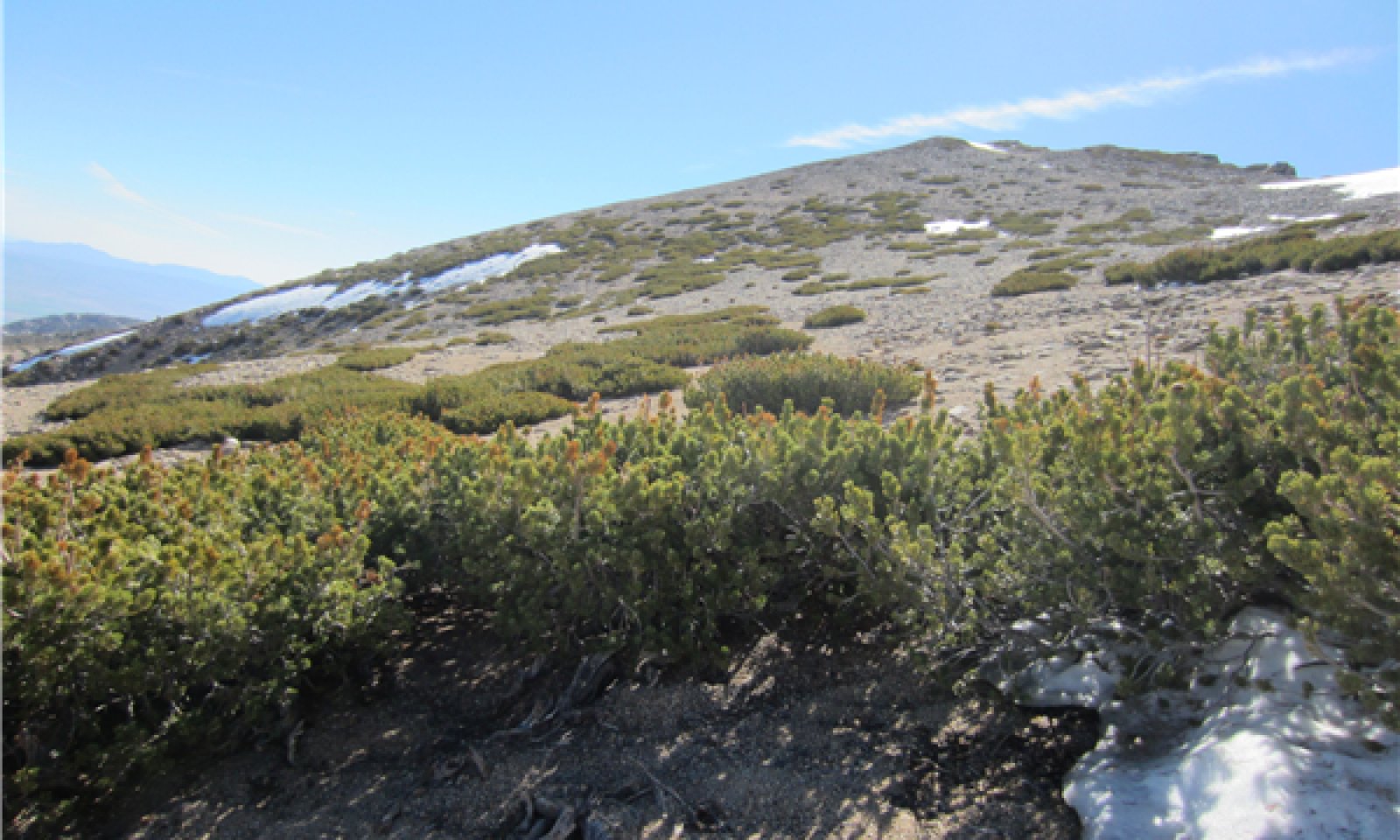

Natural Resources
Conservation Service
Ecological site R022AA201CA
Sandy Shallow Alpine Mountain Slopes
Accessed: 12/22/2024
General information
Approved. An approved ecological site description has undergone quality control and quality assurance review. It contains a working state and transition model, enough information to identify the ecological site, and full documentation for all ecosystem states contained in the state and transition model.
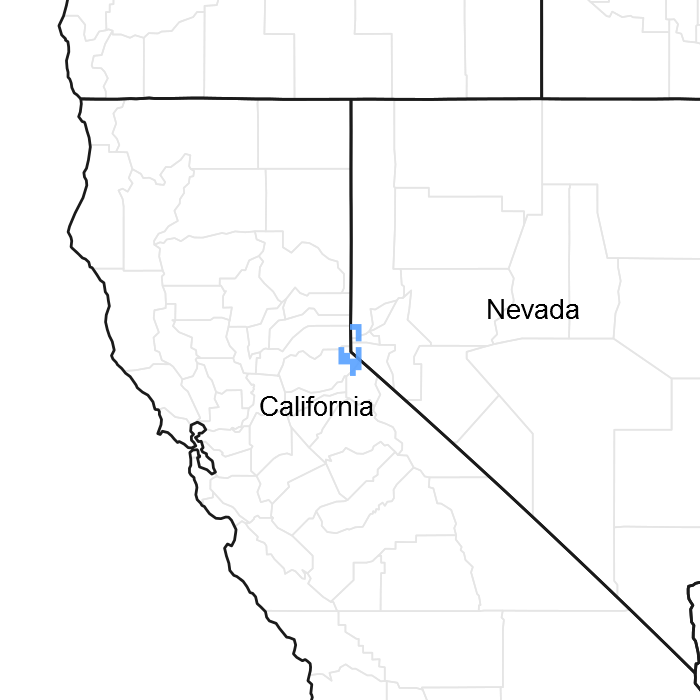
Figure 1. Mapped extent
Areas shown in blue indicate the maximum mapped extent of this ecological site. Other ecological sites likely occur within the highlighted areas. It is also possible for this ecological site to occur outside of highlighted areas if detailed soil survey has not been completed or recently updated.
MLRA notes
Major Land Resource Area (MLRA): 022A–Sierra Nevada and Tehachapi Mountains
Major Land Resource Area 22A, Sierra Nevada Mountains, is located predominantly in California and a small section of western Nevada. The area lies completely within the Sierra Nevada Section of the Cascade-Sierra Mountains Province. The Sierra Nevada range is highly asymmetrical with a long, gentle western slope, and a very abrupt eastern slope. The Sierra Nevada consists of hilly to steep mountains and occasional flatter mountain valleys. Elevation ranges between 1,500 and 9,000 ft throughout most of the range, but certain peaks often exceed 12,000 ft. The highest point in the continental US occurs in this MLRA (Mount Whitney, 14,494 ft). Most of the Sierra Nevada is dominated by granitic rock of the Mesozoic age, known as the Sierra Nevada Batholith. The northern half is flanked on the west by a metamorphic belt, which consists of highly metamorphosed sedimentary and volcanic rocks of Paleozoic and Mesozoic ages. Additionally, glacial activity of the Pleistocene has played a major role in shaping Sierra Nevada features, including cirques, arêtes, and glacial deposits and moraines. Average annual precipitation ranges from 20 to 80 inches in most of the area, with increases along elevational and south-north gradients. Soil temperature regime ranges from mesic, frigid, and cryic. Due to the extreme elevational range found within this MLRA, Land Resource Units (LRUs) were designated to group the MLRA into similar land units.
LRU "A" Sierran Alpine: This LRU occurs at the highest elevations of the Sierra Nevada, above treeline on upper mountain slopes, exposed ridges and mountain peaks. This LRU begins at the alpine treeline ecotone, which occurs at approximately 11,000 feet in the south and central areas and approximately 9,800 feet in the north, and extends up to 14,494 feet (Mount Whitney). Mean annual air temperature (MAAT) ranges from 29 to 35 degrees F, mean annual precipitation (MAP) ranges from 39 to 60 inches, and the frost free season is 20 to 30 days.
Ecological site concept
This ecological site occurs in the lowest elevations of the alpine LRU, at the alpine treeline ecotone, typically between 10,000 and 11,000 feet, on mountain slopes typically ranging from 8 to 30 percent. Soils are derived from granitic parent material, and are shallow over paralithic granitic bedrock, with a sandy skeletal particle size class. Patches of krummholz whitebark pine (Pinus albicaulis) dominate. Wind exposure, cold temperatures, and a short growing season, limit the growth of whitebark pine to a krummholz growth form. Coarse soils with very low water holding capacity support a minimal but unique component of dwarf alpine plants.
Associated sites
F022AC001CA |
Cryic Sandy Mountain Slopes This ecological site occurs at lower elevations and in more sheltered conditions than this ESD. Whitebark pine woodlands are present. |
---|---|
R022AC204CA |
Cryic, Umbric Or Andic Slopes This ecological site occurs soils with an umbric horizon, or andic parent materials. Mountain sagebrush (Artemisia tridentata ssp. vasayana) and antelope bitterbrush (Purshia tridentata) are dominant. |
Similar sites
R022AA101CA |
Sandy Very Deep Alpine Mountain Slopes This ecological site occurs on very deep soils in the southern Sierra Nevada. It is dominated by krummholtz whitebark pine. |
---|
Table 1. Dominant plant species
Tree |
Not specified |
---|---|
Shrub |
(1) Pinus albicaulis |
Herbaceous |
(1) Arabis |
Physiographic features
This site is found on mountain slopes with elevation ranging from 9,000 and 11,000 feet, but the best representation of this site occurs between 10,000 and 10,800 feet. This site is found on all aspects. Slopes range from 8 to 50 percent, but are typically between 8 and 30 percent.
Table 2. Representative physiographic features
Landforms |
(1)
Mountain slope
|
---|---|
Flooding frequency | None |
Ponding frequency | None |
Elevation | 9,000 – 11,000 ft |
Slope | 4 – 50% |
Aspect | Aspect is not a significant factor |
Climatic features
The climate of this ecological site is characterized by cool temperatures, wet winters with most precipitation falling as snow in winters, and relatively dry summers. The mean annual precipitation ranges from 40 to 55 inches. The mean annual temperature ranges from 34 to 37 degrees F. The frost-free (>32F) season is 20 to 30 days. The freeze-free (>28F) season is 30 to 45 days.
There are no representative climate stations for this ecological site so maximum and minimum monthly climate data for this ESD were generated using PRISM data (PRISM Climate Group, Oregon State University, http://prism.oregonstate.edu, created 4 Feb 2004.) and the ArcGIS ESD extract tool.
Table 3. Representative climatic features
Frost-free period (average) | 37 days |
---|---|
Freeze-free period (average) | 25 days |
Precipitation total (average) | 48 in |
Figure 2. Monthly precipitation range
Figure 3. Monthly average minimum and maximum temperature
Figure 4. Annual precipitation pattern
Figure 5. Annual average temperature pattern
Influencing water features
This site is not influenced by water features.
Soil features
The soils associated with this ecological site are shallow, and formed in colluvium and residuum derived from granitic rock. They are somewhat excessively drained with rapid permeability. The soil moisture regime is xeric and the soil temperature regime is cryic. Surface rock fragments smaller than 3 mm in diameter average 25 percent cover, and larger fragments average 20 percent. Surface texture is very gravelly loamy coarse sand. Subsurface textures are very gravelly loamy coarse sand, and very gravelly coarse sand. Subsurface rock fragments smaller than 3 mm in diameter average 32 percent by volume, and larger fragments average 12 percent (for a depth of 0 to 20 inches). The Jobsis soils are correlated to this ecological site. They are Sandy-skeletal, mixed, shallow, Typic Cryorthents. Decomposed bedrock (grus) is bedrock at 10 to 20 inches.
This ecological site has been correlated to the following mapunits and soil components in the Lake Tahoe Basin Soil Survey (CA693):
Mapunit; Mapunit name; Component; Phase; Percent
CA693;9411;Freelpeak-Windyridge-Rock outcrop complex, 15 to 75 percent slopes;Jobsis;;8
CA693;9461;Whittell-Jobsis-Rock outcrop complex, cool, 30 to 75 percent slopes;Jobsis;;4
Table 4. Representative soil features
Parent material |
(1)
Colluvium
–
granite
|
---|---|
Surface texture |
(1) Very gravelly loamy coarse sand |
Family particle size |
(1) Sandy |
Drainage class | Somewhat excessively drained |
Permeability class | Rapid |
Soil depth | 10 – 20 in |
Surface fragment cover <=3" | 25% |
Surface fragment cover >3" | 20% |
Available water capacity (0-40in) |
0.6 – 1 in |
Calcium carbonate equivalent (0-40in) |
Not specified |
Electrical conductivity (0-40in) |
6 mmhos/cm |
Sodium adsorption ratio (0-40in) |
Not specified |
Soil reaction (1:1 water) (0-40in) |
4.5 – 5.5 |
Subsurface fragment volume <=3" (Depth not specified) |
32% |
Subsurface fragment volume >3" (Depth not specified) |
12% |
Ecological dynamics
Abiotic Factors:
This ecological site occurs at the alpine treeline ecotone, typically between 10,000 and 11,000 feet, on mountain slopes. Wind exposure, cold temperatures, and a short 20 to 30 day growing season, limit the growth of whitebark pine to a shrubby krummholz stature. The whitebark pine krummholz canopy is typically less than less than 6 feet in tall, and is more often 2 to 3 feet tall. The soils associated with this ecological site are shallow with very gravelly coarse loamy sand surface textures, which have very low water holding capacity, and support a minimal but unique component of dwarf alpine plants. Whitebark pine roots can penetrate deeply into the soft decomposed granitic bedrock to access additional moisture.
Ecology-Disturbance Factors:
Individual whitebark pine trees are very slow growing, and may be 1500 years old (Millar 2014). The caching of whitebark pine seeds by Clark’s nutcracker is the primary mode of seed dispersal. The birds often cache seeds in open areas that are suitable for young seedlings. If all seeds are not consumed, they give rise to dense clusters of genetically similar whitebark pine. These clusters appear to be one tree with many stems, but are more often individual trees. (Burns et al. 1990, Tomback et al. 2001a). Whitebark pine has ongoing recruitment from Clark’s nutcracker in the absence of disturbance, thus over time stand density increases.
Fire and avalanche are the primary natural drivers for succession, but this ecological site is relatively stable. Fire ignition is frequent on these exposed ridges and mountain peaks, but there is minimal and discontinuous fuel to carry large or hot fires. Small fires may play a minor role in maintaining openings that favor the germination and survival of young whitebark pine seedlings (Burns et al. 1990, Tomback et al. 2001b, Howard 2002).
Avalanche is common among the alpine peaks and ridges, and can remove swaths of vegetation in avalanche prone chutes or below wind formed cornices. However, the removal of krummholz whitebark pine by avalanche in this ecological site is unlikely or infrequent due to the low stature of the trees, and the position of this ecological site on ridges and peaks.
Whitebark pine forests are threatened by the non-native white pine blister rust (Cronartium ribicola), and the native mountain pine beetle (Dendroctonus ponderosae) (Cox 2000, Tomback et al. 2001b, Howard 2002). Severe epidemics have killed large areas of forest in the Rocky Mountains, but the whitebark pine forests in the Sierra Nevada have not suffered as high mortality. There is a complex interaction between mountain pine beetle outbreaks, white pine blister rust (WPBR) infection rates, and climate. Mountain pine beetles prefer larger diameter trees, and attack at the warmer, lower elevation zone of whitebark pine. Thus, this krummholz whitebark pine ecological site is less susceptible to mountain pine beetle attacks. White pine blister rust will infest all whitebark pines, regardless of age or elevation (Cluck 2014). Younger trees are killed quickly, once the cancer girdles the tree. Older trees typically die slower as upper cone bearing limbs are slowly girdled by the cankers.
At this time, WPBR has varying rates of infection of whitebark pine in the Sierra Nevada, with several severe infestations in the Lake Tahoe region. The non-native WPBR was introduced into North America near Vancouver, British Columbia around 1910, and has been slowly spreading across the western United States and Canada. It was first detected in the southern Sierra Nevada Mountains in 1960’s. It has not been detected on whitebark pine in the southern extent of the Sierra Nevada, but has been found on a whitebark pine in Yosemite National Park and a high Sierra location on the western slopes of the Sierra National Forest (Maloney 2011). In order for WPBR to infect whitebark pine several synchronous phenological and environmental factors need to occur. For infection to occur in five-needled white pines, relative humidity has to be greater than 90 percent, temperatures have to be between 35.6 and 64.4 degrees F (2 to 18 degrees C), and stomates need to be open to allow the WPBR entry (Maloney 2011). The basidiospores, which infect whitebark pine, are released in fall from the alternate hosts gooseberry or currents (Ribes sp.), or less commonly, lousewort or Indian paintbrush (Pedicularis or Castilleja sp.). These spores do not travel far or last long in the environment. Whitebark pine may have early onset of winter dormancy, and the stomates may be closed at the time WPBR basidiospores are released (Maloney 2011). The onset of winter dormancy is dependent upon the length of growing season, precipitation and soil water holding capacity. This ecological site occurs on shallow, coarse textured soils on upper mountains slopes. The water drains rapidly out of the root zone, so the trees on this site may go into winter dormancy even earlier than whitebark pine on finer textures soils or cooler aspects, making them less susceptible to WPBR infestation.
WPBR affects the crown and cone producing limbs of mature trees, reducing cone production, and can kill younger trees within a year. The decrease in cone production and high mortality of young trees threatens the regenerative success of this species (Maloney et al. 2012). A few studies have been conducted on genetic resistance, and results vary from no resistance (Maloney, personal communication), and 26 to 47 percent in the Rocky Mountains and the Pacific Northwest (Keane et al. 2012).
Reduced seed production affects the presence and abundance of the Clark’s nutcracker, and in return the number and distribution of seed cache (Tomback and Resler 2007, Keane et al. 2012). This can lead to recruitment below the threshold required to sustain populations (McKinney et al. 2009).
Predictions about climate change due to global warming suggest that the whitebark pine communities in the Sierra Nevada Mountains may be threatened by rising temperatures and precipitation changes. Recent California based climate models predict a 9 degree F increase in temperature by 2100, and older models predict a 2 to 4 degree F increase in winter and 4 to 8 degree increase in summer (Safford et al. 2012). Models are more variable for precipitation, but recent models for the Sierra Nevada, predict similar to slightly less precipitation. Most models agree that summers will become drier, since more of the precipitation is predicted to come as rain, and snow melt-off will occur earlier in spring (Hayhoe et al. 2004, Safford et al. 2012). Presently a severe drought is occurring in the Sierra Nevada, with 10 to 30 percent of average precipitation and very little snow accumulation. Whether this is climate driven, and thus will become more of the future normal remains to be seen.
The alpine zone is defined as having mean annual summer temperatures of less than 43.5 Degrees F or (6.4 degrees Celcius), and a growing season of less than 94 days (Korner et al. 2011). The warming temperatures will affect the structure and distribution of the alpine ecotone. High elevation areas with suitable soils and landforms for the upward migration of whitebark pine will be important for the sustainability of this community. The long lived whitebark pine will most likely persist through the initial shift in climate, but the slow regeneration of this species in the limited expanses of suitable higher elevation habitat may be slow. The intermediate affect of warming temperatures may allow for more upright tree growth of whitebark pine and increased leader growth on compact krummholz forms. Indeed, upright tree growth forms have already significantly increased in alpine krummholz whitebark pine communities (Millar et al. 2004). However, a decrease in snow pack or earlier melt off may cause dieback of leaders and exposed krummholz, creating a more compact or less extensive krummholz community.
The dwarf alpine herbaceous community may also be impacted by warming temperatures. These
small forbs and grasses lie close to the surface to obtain the maximum heat possible at this elevation. They live in a much warmer microclimate than trees would be with their limbs exposed to air currents. The krummholz structure is also formed in part by the warmer temperatures near the soil surface. A few degrees of warming may make the temperatures too extreme for these alpine species. A modeling analysis of warming temperature on the distribution of alpine forbs in the White Mountains California, predicts local extinction of several species. Fewseed drab (Draba oligosperma), a common plant on this site, is predicted to be locally extinct in the White Mountains with 3 degrees of warming (Van de Ven et al. 2007). Because the peaks are not as high where this ecological site is found, these species have less room for upward migration.
Warmer temperatures have shifted the thermal zone for mountain pine beetles upslope, subjecting higher elevations of whitebark pine to beetle attacks (Craig 2010, Keane et al. 2012, Keane and al 2013). Growth rate of whitebark pine stems has nearly doubled since 1880, due to warmer temperatures (Millar et al. 2004). The new leaders may obtain sufficient size (greater than 6 inch dbh), to be targeted by mountain pine beetles.
The historic temperature range for this ecological site is between 20 to 35 degrees F. With a 2 to 6 degree warming, species such as Sierra lodgepole pine (Pinus contorta var. murrayana), or mountain hemlock (Tsuga mertensiana) may move into this zone. A 9 degree warming shift over the next 85 years could make conditions favorable for upper montane species to establish. Species such as Jeffrey pine (Pinus jeffreyi) and California red fir (Abies magnifica) could survive with the longer growing season and warmer temperatures for seedling germination and leader growth. If lower elevation conifers establish in the whitebark pine zone, whitebark pine may become a seral species, dependent upon fire for continued regeneration and elimination of competitors.
The reference state consists of the most successionally advanced community phase (numbered 1.1) as well as other community phases that result from natural and human disturbances. Community phase 1.1 is deemed the phase representative of the most successionally advanced pre-European plant/animal community including periodic natural surface fires that influenced its composition and production. This phase is determined from the oldest modern day remnant forests and/or historic literature.
All tabular data listed for a specific community phase within this ecological site description represent a summary of one or more field data collection plots taken in communities within the community phase. Although such data are valuable in understanding the phase (kinds and amounts of ground and surface materials, canopy characteristics, community phase overstory and understory species, production and composition, and growth), it typically does not represent the absolute range of characteristics nor an exhaustive listing of species for all the dynamic communities within each specific community phase.
State and transition model
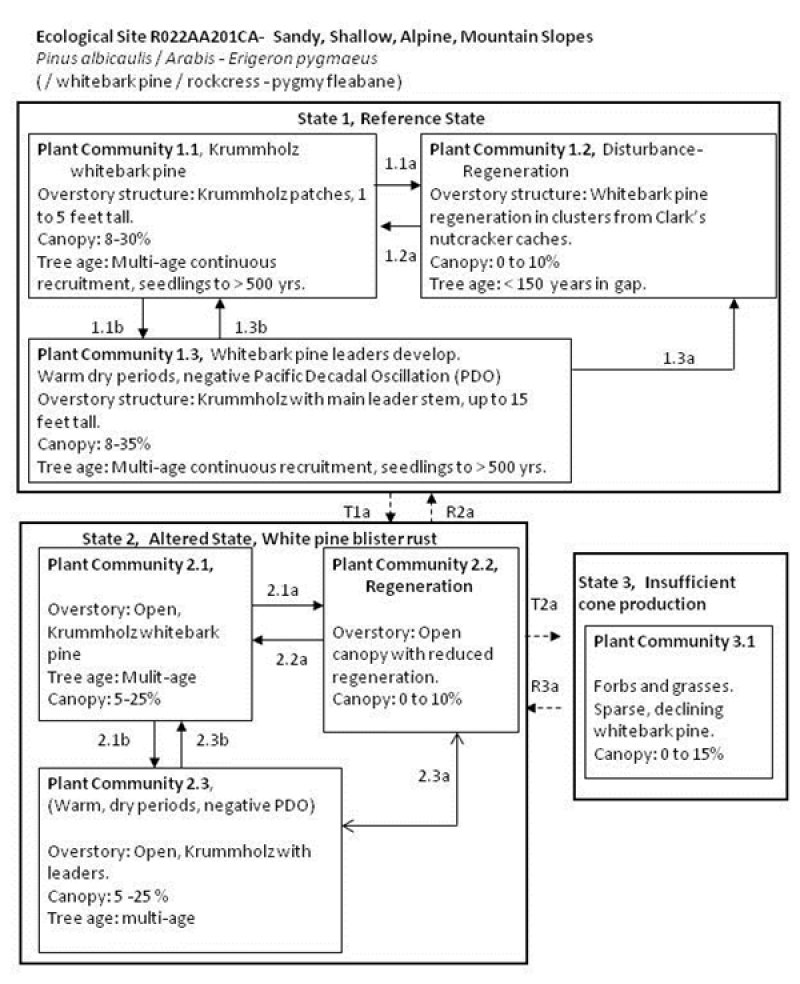
Figure 6. R022AA201CA STM
More interactive model formats are also available.
View Interactive Models
More interactive model formats are also available.
View Interactive Models
Click on state and transition labels to scroll to the respective text
State 1 submodel, plant communities
State 2 submodel, plant communities
State 3 submodel, plant communities
State 1
Reference State
This state represents the reference conditions for this ecological site. This state occurs in stands of whitebark pine where WPBR is not evident. The majority of this ecological site presently exists in this state.
Community 1.1
Krummholz whitebark pine
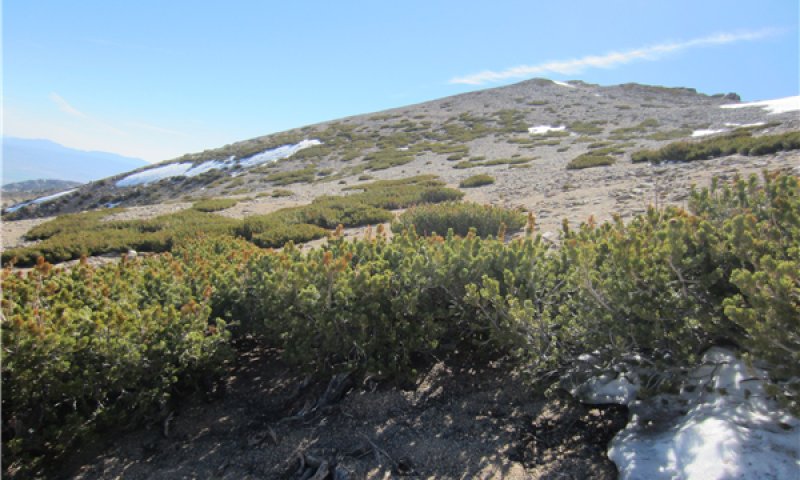
Figure 7. Krummholz Whitebark Pine on Freel Peak
The formation of krummholz whitebark pine is driven by low minimum temperatures and by wind and ice pruning of branches exposed above the snow pack. Krummholz whitebark pine typically has 8 to 30 percent cover across the slope, but within patches, cover is typically 80 to 90 percent. Canopy height can range from 2 to 6 feet, and increases down slope and in more sheltered areas. Although relatively uniform in appearance, there is variation in age due to past disturbances, micro-features that allow for more rapid growth, and constant but slow regeneration. Krummholz trees may be greater than 1,500 years old (Millar 2014), but are typically less than 800 years, and continuously develop seedlings from seed caches. Regeneration can occur within the stand, which increases basal area and canopy cover. The herbaceous community is sparse, with 1 to 5 percent total cover. Vegetation is barely noticeable from a distance, as it is low growing, compact, and interwoven among the surface gravels. Common species include, rockcress (Arabis sp.), dwarf alpine Indian paintbrush (Castilleja nana), fewseed drab (Draba oligosperma), fleabane (Erigeron spp.), cushion buckwheat (Eriogonum ovalifolium), pygmy fleabane (Erigeron pygmaeus), rosy buckwheat (Eriogonum rosense), granite prickly phlox (Linanthus pungens), Davidson''s penstemon (Penstemon davidsonii), dwarf phlox (Phlox condensata), Nevada podistera (Podistera nevadensis), Shasta knotweed (Polygonum shastense), and Watson''s spikemoss (Selaginella watsonii).
Figure 8. Annual production by plant type (representative values) or group (midpoint values)
Table 5. Annual production by plant type
Plant type | Low (lb/acre) |
Representative value (lb/acre) |
High (lb/acre) |
---|---|---|---|
Tree | 100 | 130 | 160 |
Forb | 1 | 10 | 30 |
Grass/Grasslike | 0 | 2 | 10 |
Total | 101 | 142 | 200 |
Table 6. Ground cover
Tree foliar cover | 5-30% |
---|---|
Shrub/vine/liana foliar cover | 0% |
Grass/grasslike foliar cover | 0-1% |
Forb foliar cover | 0-1% |
Non-vascular plants | 0% |
Biological crusts | 0-1% |
Litter | 0-10% |
Surface fragments >0.25" and <=3" | 40-80% |
Surface fragments >3" | 5-55% |
Bedrock | 0-2% |
Water | 0% |
Bare ground | 3-30% |
Table 7. Canopy structure (% cover)
Height Above Ground (ft) | Tree | Shrub/Vine | Grass/ Grasslike |
Forb |
---|---|---|---|---|
<0.5 | – | 0% | 0-2% | 0-5% |
>0.5 <= 1 | 0-5% | – | – | – |
>1 <= 2 | 5-20% | – | – | – |
>2 <= 4.5 | 5-20% | – | – | – |
>4.5 <= 13 | 0-1% | – | – | – |
>13 <= 40 | – | – | – | – |
>40 <= 80 | – | – | – | – |
>80 <= 120 | – | – | – | – |
>120 | – | – | – | – |
Community 1.2
Disturbance Regeneration
Fire, avalanche, or localized mortality from pathogens or insects create canopy gaps for whitebark pine regeneration from seed caches. Fire frequency studies are lacking for the krummholz whitebark community in the Sierra Nevada. The mean fire return intervals for whitebark pine forest across the US range from 29 to 300 years, while moderate severity fires range from 25 to 75 years, and stand replacing fires have greater than 140 year return interval (Fryer 2002). Lightning is the main ignition source, and fires are typically small spot fires. Avalanches are common in this area, with varying frequency, size and velocities. Some avalanche chutes may have yearly or decadal avalanches in relatively confined chutes. These zones may never develop mature whitebark pine. Larger, less frequent avalanches can uproot and cause stem breakage in the avalanche path. Whitebark pine is dependent upon Clark’s nutcracker for seed dispersal. Clark’s nutcracker prefers to cache seeds in open or disturbed areas, and those that are not recovered germinate and create young tree clusters. Seed predation in normal years may be up to 97 percent, leaving few seeds for germination. Germination and seedling establishment after fire may take several years because of the high predation rate, and short dormancy period in some seeds. Years with higher summer precipitation may have higher cone yields. The cones take two years to develop. After favorable cone production cycles, there will be more seeds left by predators for germination (Fryer 2002). Whitebark pine establishment may take longer than after fire, depending upon the degree of soil and seed cache disturbance. Seeds may need to come from new seed caches. Growth of young seedlings is slow. In a typical stand, whitebark pine reaches cone maturity at 60 to 100 years (Fryer 2002), but the krummholz whitebark pine may take longer since it is in the coldest region of the whitebark pine zone.
Community 1.3
Warm dry periods
This community develops with the vertical release of whitebark pine leaders during a slightly warmer and drier climatic period and a negative Pacific Decadal Oscillation (PDO). Past episodes have occurred between 1945 and 1976 (Millar et al. 2004). This period of favorable growth enabled thick bark development on the leaders, which allowed leaders to persist through subsequent colder periods. Favorable climate conditions in the future may allow for another pulse of leader development, or additional height increase on the existing leaders.
Pathway 1.1a
Community 1.1 to 1.2
Fire, avalanche, or pathogens create small gaps in canopy.
Pathway 1.1b
Community 1.1 to 1.3
Warm, dry period allows for leader growth and whitebark pine expansion.
Pathway 1.2a
Community 1.2 to 1.1
Time, with growth and continued regeneration of whitebark pine.
Pathway 1.3b
Community 1.3 to 1.1
Cold period with lack of snow cover causes die-back of exposed leaders and branches.
Pathway 1.3a
Community 1.3 to 1.2
Disturbances such as small fires, avalanche, pathogens, or insects create openings for regeneration.
State 2
Altered State
This state has developed with the introduction of the non-native white pine blister rust.
Community 2.1
Krummholz whitebark pine
This community is similar to community 1.1, but the krummholz whitebark pine has low to moderate infection rates from white pine blister rust. There may be death of infected younger trees, and dieback of infected branches on larger trees.
Community 2.2
Disturbance Regeneration
Regeneration occurs in canopy gaps, from seeds germinating in Clark''s nutcracker caches. Overall regeneration is lower due to reduced cone production and a higher percentage of seed consumption by Clark’s nutcracker, and potential infestation and mortality of young seedlings from WPBR infection.
Community 2.3
Leader Development
Krummholz whitebark pine develops leaders which extend vertically above the krummholz canopy height during warm and dry years. Taller tree forms begin to develop. Tree density and cover increases with infilling of whitebark pine. This phase is more vulnerable to mountain pine beetle outbreaks.
Pathway 2.1a
Community 2.1 to 2.2
Fire, death from pathogens, insects and/or avalanches create canopy openings and niches for whitebark pine seed caches and regeneration.
Pathway 2.1b
Community 2.1 to 2.3
Period of warmer dry weather and negative PDO.
Pathway 2.2a
Community 2.2 to 2.1
Time, slow regeneration of uninfected seedlings.
Pathway 2.3b
Community 2.3 to 2.1
Cold winter, low snow pack, dieback of leaders due to exposure.
Pathway 2.3a
Community 2.3 to 2.2
Fire, death from pathogens, and/or avalanches create canopy openings and niches for whitebark pine seed caches and regeneration.
State 3
Insufficient Cone Production
This state occurs, when there is insufficient regeneration of whitebark pine due to WBPB infection to maintain the stand viability.
Community 3.1
Declining whitebark pine
This state has not occurred in this ecological site, but it possibly crossed into this state on nearby volcanic soils (R022AC200CA). If cone production continues to decline, and disease resistance is not found, whitebark pine will slowly decline over hundreds of years. In the absence of whitebark pine, the sparse dwarf alpine forbs and grasses may become dominant.
Transition 1a
State 1 to 2
This transition is triggered by infection of whitebark pine by Cronartium ribicola, cause of white pine blister rust (WPBR), within this ecological site. WPBR affects the crown and cone producing limbs of mature trees, reducing cone production, and can kill younger trees within a year. The decrease in cone production and high mortality of young trees threatens the regenerative success of this species (Maloney et al. 2012). Repeat waves of infection by WPBR under favorable climatic conditions can worsen the situation. Reduced seed production affects the presence and abundance of Clark’s nutcracker, and thus the number and distribution of seed caches (Tomback and Resler 2007, Keane et al. 2012). This can lead to recruitment below the threshold required to sustain populations (McKinney et al. 2009).
Restoration pathway R2a
State 2 to 1
Restoration practices that have been experimented with include spraying pesticides for mountain pine beetle, and planting of hopeful, disease resistant whitebark pine.
Transition T2a
State 2 to 3
Whitebark pine is dependent upon Clark’s nutcracker for seed dispersal. Clark’s nutcrackers cache seeds for later retrieval. Those caches that are not recovered, germinate in small clusters. WPBR, MPB or the combination of the two, can drastically reduce cone production. This transition occurs when cone production is less than 1000 cones/ Ha and basal area is < .5 m2/ acre. Below this threshold there may be insufficient seeds for dispersal by Clark’s nutcracker (McKinney et al. 2009).
Restoration pathway R3a
State 3 to 2
Restoration practices that have been experimented with include spraying pesticides for mountain pine beetle, and planting of hopeful, disease resistant whitebark pine.
Additional community tables
Table 8. Community 1.1 plant community composition
Group | Common name | Symbol | Scientific name | Annual production (lb/acre) | Foliar cover (%) | |
---|---|---|---|---|---|---|
Tree
|
||||||
1 | Trees | 100–160 | ||||
whitebark pine | PIAL | Pinus albicaulis | 100–160 | 10–30 | ||
Forb
|
||||||
2 | Forbs | 1–30 | ||||
rockcress | ARABI2 | Arabis | 0–15 | 0–2 | ||
dwarf alpine Indian paintbrush | CANA3 | Castilleja nana | 0–5 | 0–1 | ||
fewseed draba | DROL | Draba oligosperma | 0–5 | 0–1 | ||
fleabane | ERIGE2 | Erigeron | 0–5 | 0–1 | ||
cushion buckwheat | EROV | Eriogonum ovalifolium | 0–5 | 0–1 | ||
pygmy fleabane | ERPY | Erigeron pygmaeus | 0–5 | 0–1 | ||
rosy buckwheat | ERRO | Eriogonum rosense | 0–5 | 0–1 | ||
granite prickly phlox | LIPU11 | Linanthus pungens | 0–5 | 0–1 | ||
Davidson's penstemon | PEDA2 | Penstemon davidsonii | 0–5 | 0–1 | ||
dwarf phlox | PHCO11 | Phlox condensata | 0–5 | 0–1 | ||
Nevada podistera | PONE4 | Podistera nevadensis | 0–5 | 0–1 | ||
Shasta knotweed | POSH | Polygonum shastense | 0–5 | 0–1 | ||
Watson's spikemoss | SEWA2 | Selaginella watsonii | 0–5 | 0–1 | ||
Grass/Grasslike
|
||||||
3 | Grasslike | 0–10 | ||||
sedge | CAREX | Carex | 0–10 | 0–2 |
Interpretations
Animal community
The Clark’s Nutcracker and squirrels play a major role in seed dispersal for whitebark pine, by caching the seeds. Bears have been reported to raid the squirrel middens for the whitebark seeds (Howard 2002). The whitebark seeds provide valuable nutrition, and are an important food for bears, birds, and rodents. The trees also provide cover for and nesting cavities for birds and other wildlife.
Hydrological functions
The soils associated with this ecological site are in hydrologic group d. Soils in this group have high runoff potential when thoroughly wet. Water movement through the soil is restricted or very restricted. In this case, the water movement is restricted by the decomposed granite bedrock. These soils quickly absorb water when dry, but can rill or gully with excessive surface run off, or surface disturbance.
Recreational uses
Hiking and mountain biking are the main recreational activities in this area. Due to the highly erodible sandy soil, trails should be constructed carefully.
Wood products
This has very low productivity and is not suited for timber or firewood production.
Supporting information
Inventory data references
The following NRCS plots were used to describe this ecological site:
State/phase 1.1:
GWF02032
GWF02552
RX02h46- Type Location
SM02h44
SM02h48a
02CA693-010-b (photo only)
Type locality
Location 1: El Dorado County, CA | |
---|---|
UTM zone | N |
UTM northing | 4304907 |
UTM easting | 247952 |
General legal description | The type location is approximately a quarter mile West of Freel Peak, in the Lake Tahoe Basin. |
Other references
Burns, R. M., B. H. Honkala, and United States. Forest Service. 1990. Silvics of North America. U.S. Dept. of Agriculture For sale by the Supt. of Docs., U.S. G.P.O., Washington.
Cluck, D. 2014. Mountain Pine Beetle Outbreak in the Warner Mountains: Implications for Whitebark Pine.in Northern California Botanist Special Workshop / Session, Chico, CA.
Cox, S. 2000. Management of Whitebark Pine (Pinus albicaulis) in North American Forest and National Parks. Colorado State University.
Craig, R. K. 2010. “Stationarity is dead”—long live transformation: five principles for climate change adaptation law. Harvard Environmental Law Review:66.
Fryer, J. L. 2002. Pinus albicaulis. . In: Fire Effects Information System, [Online]. U.S. Department of Agriculture, Forest Service, Rocky Mountain Research Station, Fire Sciences Laboratory
Hayhoe, K., D. Cayan, C. B. Field, P. C. Frumhoff, E. P. Mauren, N. L. Miller, S. C. Moser, S. H. Schneider, K. N. Cahill, E. E. Cleland, L. Dale, R. Drapek, R. M. Hanemann, L. S. Kalkstein, J. Lenihan, C. K. Lunch, R. P. Neilson, S. C. Sheridan, and J. H. Verville. 2004. Emissions pathways, climate change, and impacts on California. Proceedings of the National Academy of Sciences 101.
Howard, J. 2002. Pinus albicaulis. Fire Effects Information System. U.S. Department of Agriculture, Forest Service, Rocky Mountain Research Station, Fire Sciences Laboratory
Keane, R. E., and e. al. 2013. Climate Change and Whitebark Pine: Compelling reasons for restoration. WPEF Climate Change White Paper.
Keane, R. E., D. F. Tomback, C. A. Aubry, A. D. Bower, E. M. Campbell, C. L. Cripps, M. B. Jenkins, M. F. Mahalovich, M. Manning, S. T. McKinney, M. P. Murray, D. L. Perkins, D. P. Reinhart, C. Ryan, A. W. Schoettle, and C. M. Smith. 2012. A Wide Range Restoration Strategy for Whitebark Pine (Pinus albicaulis). Page 108 GTR RMRS-GTR-279. US Department of Agriculture, Forest Service, Rocky Mountain Research Center, Fort Collins, CO.
Korner, C., J. Paulsen, and E. M. Spehn. 2011. A definition of mountains and their bioclimatic belts for global comparisons of biodiversity data. Alpine Botany.
Maloney, P. 2011. Incidence and distribution of white pine blister rust in the high elevation forests of California. Forest Pathology:8.
Maloney, P., D. R. Vogler, C. E. Jensen, and A. D. Mix. 2012. Ecology of whitebark pine populations in relation to white pine blister rust infection in subalpine forests of the Lake Tahoe Basin, USA: Implications for restoration. Forest Ecology and Management 280:166-175.
McKinney, S. T., C. E. Fiedler, and D. F. Tomback. 2009. Invasive pathogen threatens bird–pine mutualism: implications for sustaining a high-elevation ecosystem. Ecological Applications 19:10.
Millar, C. I. 2014. Climate, Bark Beetles, and High Elevation Pines (Whitebark and Limber) in the Great Basin: Not Always a Bad Combination. .in Northern California Botanist Special Workshop / Session, Chico, CA.
Millar, C. I., R. D. Westfall, D. L. Delaney, J. C. King, and L. J. Graumlich. 2004. Response of subalpine conifers in the Sierra Nevada, California, USA, to 20th-century warming and decadal climate variability. Arctic, Antarctic, and Alpine Research 36:181-200.
Safford, H. D., M. North, and M. D. Meyer. 2012. Climate change and the relevance of historical forest conditions. Page 22 in M. North, editor. Managing Sierra Nevada Forest. United States Department of Agriculture.
Tomback, D. F., S. F. Arno, and R. E. Keane. 2001b. Whitebark Pine Communities: Ecology & Restoration. Island Press, Washington D.C.
Tomback, D. F., and L. M. Resler. 2007. Invasive pathogens at alpine treeline: consequences for treeline dynamics. Physical Geography 28:397-418.
Van de Ven, C. M., S. B. Weiss, and W. G. Ernst. 2007. Plant Species Distributions under Present Conditions and Forecasted for Warmer Climates in an Arid Mountain Range. Earth Interactions 11:33.
Contributors
Marchel Munnecke
Rangeland health reference sheet
Interpreting Indicators of Rangeland Health is a qualitative assessment protocol used to determine ecosystem condition based on benchmark characteristics described in the Reference Sheet. A suite of 17 (or more) indicators are typically considered in an assessment. The ecological site(s) representative of an assessment location must be known prior to applying the protocol and must be verified based on soils and climate. Current plant community cannot be used to identify the ecological site.
Author(s)/participant(s) | |
---|---|
Contact for lead author | |
Date | |
Approved by | |
Approval date | |
Composition (Indicators 10 and 12) based on | Annual Production |
Indicators
-
Number and extent of rills:
-
Presence of water flow patterns:
-
Number and height of erosional pedestals or terracettes:
-
Bare ground from Ecological Site Description or other studies (rock, litter, lichen, moss, plant canopy are not bare ground):
-
Number of gullies and erosion associated with gullies:
-
Extent of wind scoured, blowouts and/or depositional areas:
-
Amount of litter movement (describe size and distance expected to travel):
-
Soil surface (top few mm) resistance to erosion (stability values are averages - most sites will show a range of values):
-
Soil surface structure and SOM content (include type of structure and A-horizon color and thickness):
-
Effect of community phase composition (relative proportion of different functional groups) and spatial distribution on infiltration and runoff:
-
Presence and thickness of compaction layer (usually none; describe soil profile features which may be mistaken for compaction on this site):
-
Functional/Structural Groups (list in order of descending dominance by above-ground annual-production or live foliar cover using symbols: >>, >, = to indicate much greater than, greater than, and equal to):
Dominant:
Sub-dominant:
Other:
Additional:
-
Amount of plant mortality and decadence (include which functional groups are expected to show mortality or decadence):
-
Average percent litter cover (%) and depth ( in):
-
Expected annual annual-production (this is TOTAL above-ground annual-production, not just forage annual-production):
-
Potential invasive (including noxious) species (native and non-native). List species which BOTH characterize degraded states and have the potential to become a dominant or co-dominant species on the ecological site if their future establishment and growth is not actively controlled by management interventions. Species that become dominant for only one to several years (e.g., short-term response to drought or wildfire) are not invasive plants. Note that unlike other indicators, we are describing what is NOT expected in the reference state for the ecological site:
-
Perennial plant reproductive capability:
Print Options
Sections
Font
Other
The Ecosystem Dynamics Interpretive Tool is an information system framework developed by the USDA-ARS Jornada Experimental Range, USDA Natural Resources Conservation Service, and New Mexico State University.
Click on box and path labels to scroll to the respective text.