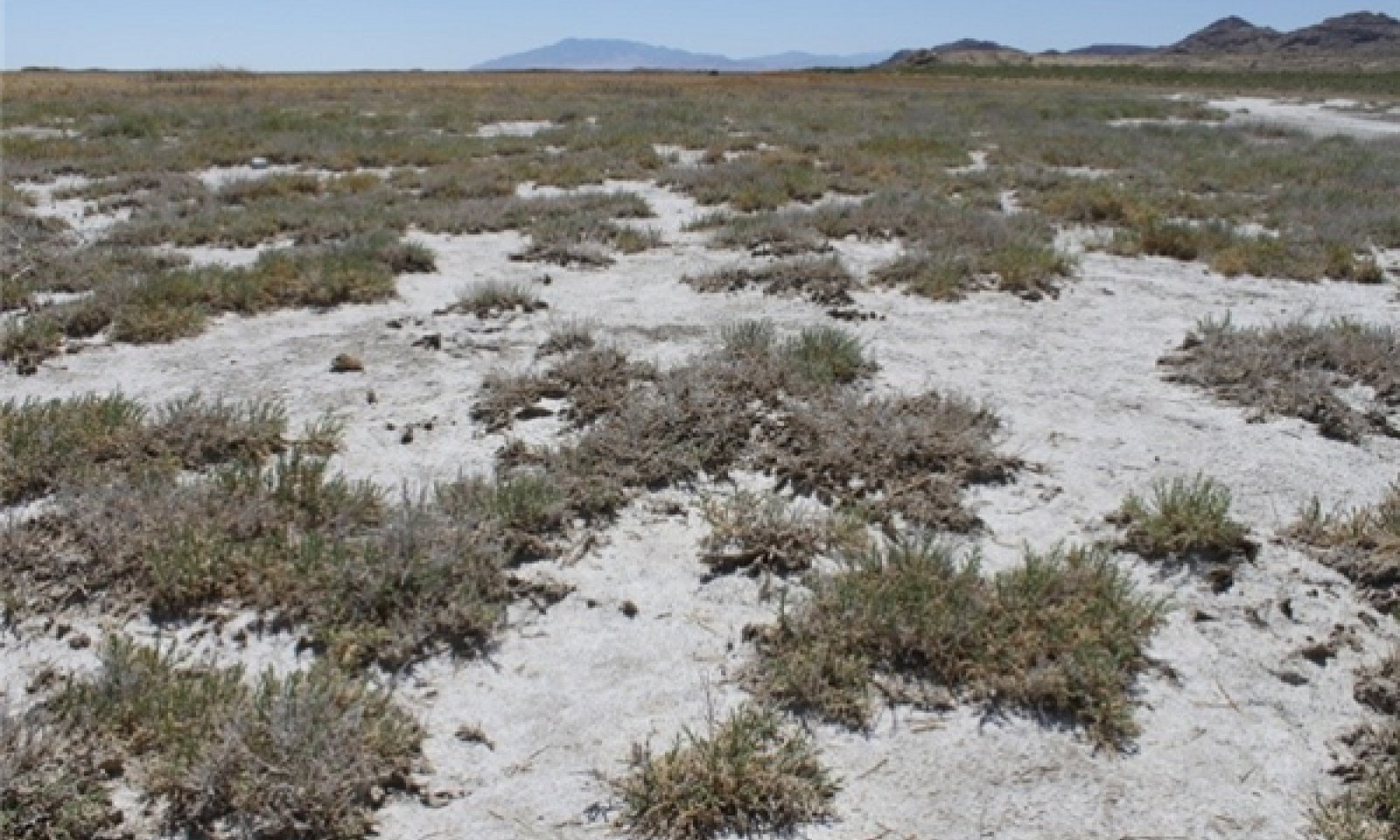

Natural Resources
Conservation Service
Ecological site R028AY009NV
SALINE FLAT
Accessed: 01/10/2025
General information
Provisional. A provisional ecological site description has undergone quality control and quality assurance review. It contains a working state and transition model and enough information to identify the ecological site.
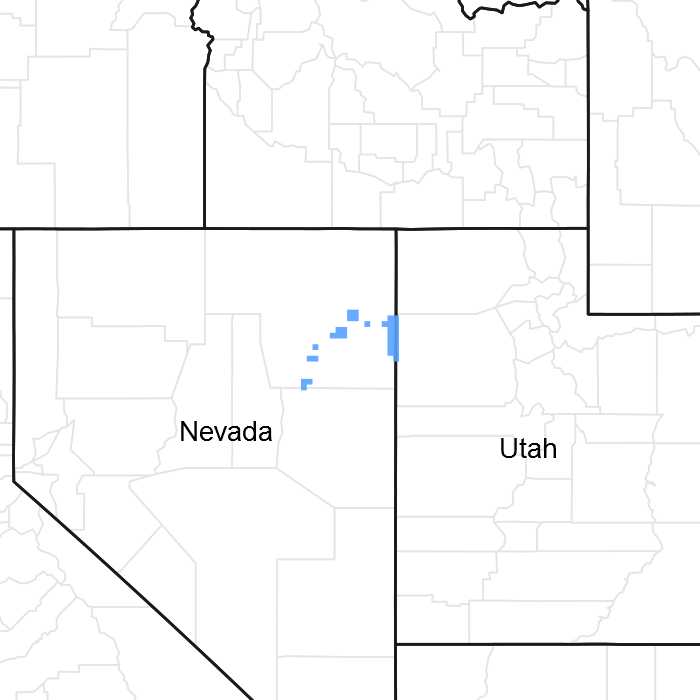
Figure 1. Mapped extent
Areas shown in blue indicate the maximum mapped extent of this ecological site. Other ecological sites likely occur within the highlighted areas. It is also possible for this ecological site to occur outside of highlighted areas if detailed soil survey has not been completed or recently updated.
MLRA notes
Major Land Resource Area (MLRA): 028A–Ancient Lake Bonneville
MLRA 28A occurs in Utah (82%), Nevada (16%), and Idaho (2%). It makes up about 36,775 square miles. A large area west and southwest of Great Salt Lake is a salty playa. This area is the farthest eastern extent of the Great Basin Section of the Basin and Range Province of the Intermontane Plateaus. It is an area of nearly level basins between widely separated mountain ranges trending north to south. The basins are bordered by long, gently sloping alluvial fans. The mountains are uplifted fault blocks with steep side slopes. They are not well dissected because of low rainfall in the MLRA. Most of the valleys are closed basins containing sinks or playa lakes. Elevation ranges from 3,950 to 6,560 ft. in the basins and from 6,560 to 11,150 ft. in the mountains. Most of this area has alluvial valley fill and playa lakebed deposits at the surface. Great Salt Lake is all that remains of glacial Lake Bonneville. A level line on some mountain slopes indicates the former extent of this glacial lake. Most of the mountains in the interior of this area consist of tilted blocks of marine sediments from Cambrian to Mississippian age. Scattered outcrops of Tertiary continental sediments and volcanic rocks are throughout the area. The average annual precipitation is 5 to 12 ins. in the valleys and is as much as 49 ins. in the mountains. Most of the rainfall occurs as high-intensity, convective thunderstorms during the growing season. The driest period is from midsummer to early autumn. Precipitation in winter typically occurs as snow. The average annual temperature is 39 to 53 °F. The freeze-free period averages 165 days and ranges from 110 to 215 days, decreasing in length with elevation. The dominant soil orders in this MLRA are Aridisols, Entisols, and Mollisols. The soils in the area dominantly have a mesic or frigid soil temperature regime, an aridic or xeric soil moisture regime, and mixed mineralogy. They generally are well drained, loamy or loamy-skeletal, and very deep.
Ecological site concept
This site occurs on lakeplains. Slopes range from 0 to 2 percent. Elevations are 4200 to 4400 feet.
Average annual precipitation is 5 to 8 inches. Mean annual air temperature is to 47 degrees F. The average growing season is about 100 to 130 days.
The soils associated with this site are very deep and poorly or very poorly drained. The surface layer of these soils is flocculated due to the extremely high salt concentration which potentially improves infiltration rates. High salt concentrations reduce the available water capacity of these soils and adversely affect seed viability and germination. The soils are saturated with water during most of the year within a depth of 40 inches. Capillary moisture from the shallow water table enhances soil moisture in that part of the soil profile below the surface layer.
The reference state is dominated by iodinebush and inland saltgrass. Production ranges from 75 to 150 pounds per acre.
Associated sites
R028AY045NV |
SODIC FLAT |
---|---|
R028AY046NV |
WET SODIC BOTTOM |
Similar sites
R028AY045NV |
SODIC FLAT SAVE4 major shrub; less productive site |
---|---|
R028BY020NV |
SODIC FLAT 5-8 P.Z. SAVE4 dominant shrub; more productive site |
R028AY026NV |
SODIC FLOODPLAIN SPAI dominant plant; more productive site |
Table 1. Dominant plant species
Tree |
Not specified |
---|---|
Shrub |
(1) Allenrolfea occidentalis |
Herbaceous |
(1) Distichlis spicata |
Physiographic features
This site occurs on lake plains. Slopes range from 0 to 2 percent. Elevations are 4200 to 4400 feet.
Table 2. Representative physiographic features
Landforms |
(1)
Lake plain
|
---|---|
Flooding duration | Very brief (4 to 48 hours) |
Flooding frequency | Rare |
Ponding duration | Very brief (4 to 48 hours) to brief (2 to 7 days) |
Ponding frequency | None to rare |
Elevation | 4,200 – 4,400 ft |
Slope | 2% |
Ponding depth | 2 in |
Aspect | Aspect is not a significant factor |
Climatic features
Nevada’s climate is predominantly arid, with large daily ranges of temperature, infrequent severe storms, heavy snowfall in the higher mountains, and great location variations with elevation. Three basic geographical factors largely influence Nevada’s climate: continentality, latitude, and elevation. Continentality is the most important factor. The strong continental effect is expressed in the form of both dryness and large temperature variations. Nevada lies on the eastern, lee side of the Sierra Nevada Range, a massive mountain barrier that markedly influences the climate of the State. The prevailing winds are from the west, and as the warm moist air from the Pacific Ocean ascend the western slopes of the Sierra Range, the air cools, condensation occurs and most of the moisture falls as precipitation. As the air descends the eastern slope, it is warmed by compression, and very little precipitation occurs. The effects of this mountain barrier are felt not only in the West but throughout the state, with the result that the lowlands of Nevada are largely desert or steppes. The temperature regime is also affected by the blocking of the inland-moving maritime air. Nevada sheltered from maritime winds, has a continental climate with well-developed seasons and the terrain responds quickly to changes in solar heating.
Nevada lies within the mid-latitude belt of prevailing westerly winds which occur most of the year. These winds bring frequent changes in weather during the late fall, winter and spring months, when most of the precipitation occurs. To the south of the mid-latitude westerlies, lies a zone of high pressure in subtropical latitudes, with a center over the Pacific Ocean. In the summer, this high-pressure belt shifts northward over the latitudes of Nevada, blocking storms from the ocean. The resulting weather is mostly clear and dry during the summer and early fall, with scattered thundershowers. The eastern portion of the state receives significant summer thunderstorms generated from monsoonal moisture pushed up from the Gulf of California, known as the North American monsoon. The monsoon system peaks in August and by October the monsoon high over the Western U.S. begins to weaken and the precipitation retreats southward towards the tropics (NOAA 2004).
Average annual precipitation is 5 to 8 inches. Mean annual air temperature is to 47 degrees F. The average growing season is about 100 to 130 days.
Mean annual precipitation at Wendover WSO Airport, Utah climate station (429382)is 4.59 inches.
Monthly mean precipitation is:
January 35.8; February 42.9; March 53.1;
April 62.5; May 72.7; June 82.9; July 92.5;
August 89.9; September 78.9; October 63.7;
November 47.6; December 37.
Table 3. Representative climatic features
Frost-free period (average) | 115 days |
---|---|
Freeze-free period (average) | 0 days |
Precipitation total (average) | 7 in |
Figure 2. Monthly average minimum and maximum temperature
Figure 3. Annual precipitation pattern
Figure 4. Annual average temperature pattern
Influencing water features
This site receives run-in moisture from adjacent sites and may pond after summer convection storms or during snow melt.
Soil features
The soils associated with this site are very deep and poorly drained to very poorly drained. The surface layer of these soils is flocculated due to the extremely high salt concentration which potentially improves infiltration rates. High salt concentrations reduce the available water capacity of these soils and adversely affect seed viability and germination. The soils are strongly saline and strongly alkaline. The soils are saturated with water during most of the year within a depth of 40 inches. Capillary moisture from a shallow water table enhances soil moisture in that part of the soil profile below the surface layer. Runoff is neglibile if ponded to very high if otherwise. Flooding and ponding may occur in some areas for short periods. Potential for sheet and rill erosion is slight. The soil temperature regime is mesic and the soil moisture regime is aquic during seasonal periods of saturation and reduction. Soil series associated with this site include: Saltair.
The representative soil series is Saltair, a Fine-silty, mixed, superactive, mesic Typic Aquisalids. Diagnostic horizons include an ochric epipedon from the soil surface to 1 inch, and a salic horizon from the soil surface to 60 inches. Clay content in the particle control sections average 20 to 35 percent. Reaction is moderately alkaline or very strongly alkaline. Effervescence is strongly effervescent. Lithology consists lacustrine deposits and alluvium from limestone, shale, and quartzite.
Table 4. Representative soil features
Parent material |
(1)
Lacustrine deposits
–
limestone
(2) Alluvium – shale (3) Alluvium – quartzite |
---|---|
Surface texture |
(1) Silt loam (2) Silty clay loam |
Family particle size |
(1) Loamy |
Drainage class | Poorly drained to very poorly drained |
Permeability class | Slow to very slow |
Soil depth | 60 – 84 in |
Surface fragment cover <=3" | Not specified |
Surface fragment cover >3" | Not specified |
Available water capacity (0-40in) |
6.6 – 6.7 in |
Calcium carbonate equivalent (0-40in) |
15 – 30% |
Electrical conductivity (0-40in) |
50 – 250 mmhos/cm |
Sodium adsorption ratio (0-40in) |
70 – 1,000 |
Soil reaction (1:1 water) (0-40in) |
8.2 – 9 |
Subsurface fragment volume <=3" (Depth not specified) |
Not specified |
Subsurface fragment volume >3" (Depth not specified) |
Not specified |
Ecological dynamics
An ecological site is the product of all the environmental factors responsible for its development and it has a set of key characteristics that influence a site’s resilience to disturbance and resistance to invasives. Key characteristics include 1) climate (precipitation, temperature), 2) topography (aspect, slope, elevation, and landform), 3) hydrology (infiltration, runoff), 4) soils (depth, texture, structure, organic matter), 5) plant communities (functional groups, productivity), and 6) natural disturbance regime (fire, herbivory, etc.) (Caudle et al 2013). Biotic factors that influence resilience include site productivity, species composition and structure, and population regulation and regeneration (Chambers et al. 2013).
The Great Basin shrub communities have high spatial and temporal variability in precipitation, both among years and within growing seasons. Nutrient availability is typically low but increases with elevation and closely follows moisture availability. The moisture resource supporting the greatest amount of plant growth is usually the water stored in the soil profile during the winter. The invasibility of plant communities is often linked to resource availability. Disturbance can decrease resource uptake due to damage or mortality of the native species and depressed competition or can increase resource pools by the decomposition of dead plant material following disturbance.
These salt-desert shrub communities are dominated by phreatophytes that depend for their water supply upon groundwater that lies within reach of their roots. The Saline Flat site is characterized by unvegetated playa areas interspersed with small vegetated mounds dominated by iodinebush and inland saltgrass. The plants on this site are halophytes and are highly tolerant of saline soil conditions. Halophytes tend to be smaller in size in areas with concentrated salt, and larger in less saline conditions (Flowers 1934). Halophyte seeds remain viable for long periods of time under saline conditions and tend to germinate in the spring when salt concentrations are reduced due to precipitation (Unger 1982, Gul and Weber 1999, Khan et al 2000). Blank et al (1998) studied an area with iodinebush on mounds similar to this site and found that the mounds seemed to form periodically; mounds had mature vegetation but were too saline to allow for recruitment. It was hypothesized that when mature plants die, mounds tend to erode. Only upon new mound formation would these species be able to germinate.
Iodinebush is a chenopod and a C3-pathway halophytic plant that grows in the arid environment of the Western United States where halomorphic soil induces extreme osmotic stress with erratic and low precipitation during the growing period. Iodinebush is generally found on eolian mounds at the margins of salt marshes and salt playas (Trent et al 1997, Gul and Weber 1999). These mounds average 0.3 meters in height and appear to be favorable for plant recruitment and survivorship, however this value is lost after a number of years because of accumulation of salts (Blank et al 1998). Iodinebush grows well in soils with 6% sodium chloride (NaCl). It is one of the most salt tolerant species in salt playas of the Great Basin. The dry summer season allows for salt to accumulate on the soil surface which prevents most plants from growing, except for iodinebush (Weber et al. 2002). Iodinebush roots were extracted from a mound and found that roots extended over 10 meters into the unvegetated playa (Blank et al 1998). The diameter of larger roots was over 5 cm, and many had between 90 and 120 growth rings, indicating that iodinebush is quite long-lived. Iodinebush relies on a persistant seedbank that can remain dormant until salinity stress is alleviated (Gul and Weber 2001). The large taproot of iodinebush can reach water table depths to 25 feet (Meinzer 1927).
Saltgrass is a C4-pathway dioecious warm-season perennial rhizomatous grass that is highly adapted to saline areas (Comstock and Eleringer 1992, Newman and Gates 2000). Marcum and Kopec (1997) found saltgrass more tolerant of increased levels of salinity than alkaline sacaton, therefore dewatering and/or long-term drought causing increased levels of salinity would create environmental conditions more favorable to saltgrass over alkali sacaton. Saltgrass is a classified as a phreatophyte and a facultative wetland species. It occurs where water table depths are from the soil surface to 10 or more feet (Meinzer 1927).
Alkali sacaton is a long-lived, warm-season, densely tufted perennial bunchgrass. It is considered a phreatophyte and a facultative wetland species in this region (USDA, NRCS 2015). Alkali sacaton has deep, coarse roots allowing it reach water tables at depths from 4 to 27 feet (Meinzer 1927). It reproduces from seeds and tillers and is a prolific seed producer. The seeds remain viable for several years because of the hard, waxy seed coats (USDA-Forest Service 1988).
The ecological site has low resilience to disturbance and resistance to invasion. Fire is not a typical disturbance in these sparsely vegetated communities. Changes in hydrology will affect plant communities; for example, lowering of the water table will decrease the herbaceous understory and eventually affect shrub species as well. If the soils in this site are excessively disturbed, soil blowouts followed by ponding may lead to the formation of small playettes and loss of vegetation for a time. There is a greater risk of this occurring in areas with high off-highway vehicle (OHV) use. It has been observed that soil disturbance appeared to enable vegetation recruitment (Blank et al 1998), but no further research has verified this. In the presence of non-native invasive weeds, soil disturbance could allow for further invasion. The introduction of annual weedy species, like halogeton, may cause an increase in fire frequency and eventually lead to an annual state. Two possible alternative stable states have been identified for this site.
Fire Ecology:
Historically, salt-desert shrub communities had sparse understories and bare soil in intershrub spaces, making these communities somewhat resistant to fire (Young 1983, Paysen et al. 2000). They may burn only during high fire hazard conditions; for example, years with high precipitation can result in almost continuous fine fuels, increasing fire hazard (West 1994, Paysen et al. 2000).
Loren and Kadlec (1985) found that fire followed by flooding one week later eliminated saltgrass from a Utah salt marsh; they found that although fire did not kill the plant’s rhizomes, the grass was not able to recover after flooding. Without immediate flooding, saltgrass may actually increase in cover after fire (de Szalay and Resh 1997).
Alkali sacaton is tolerant of fire, but can be killed by severe fire. Summer fires are more detrimental than winter fires. Recovery is typically two to four years (Newman and Gates 2000).
State and transition model
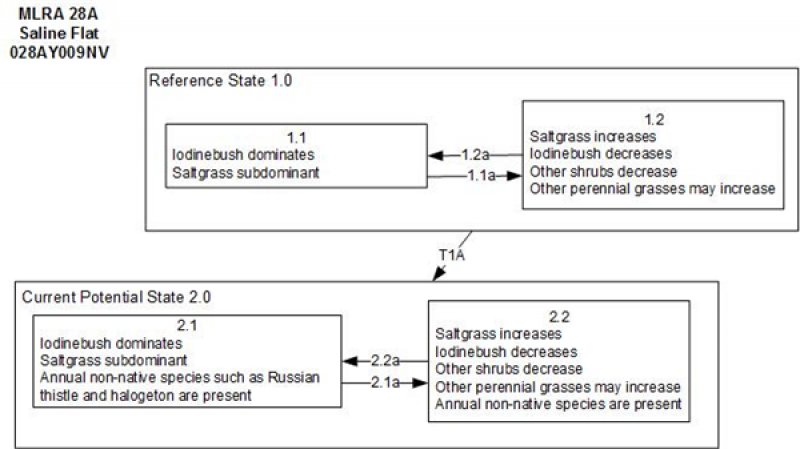
Figure 5. State and Transition Model
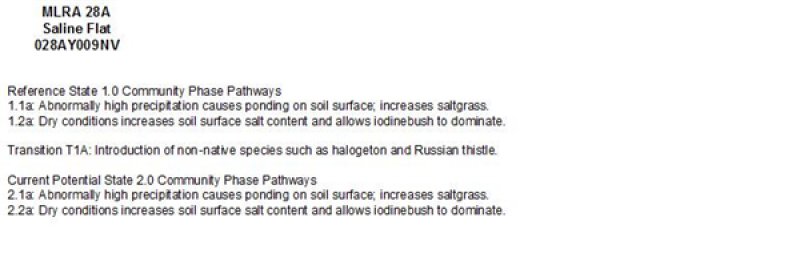
Figure 6. Legend
More interactive model formats are also available.
View Interactive Models
More interactive model formats are also available.
View Interactive Models
Click on state and transition labels to scroll to the respective text
Ecosystem states
State 1 submodel, plant communities
State 2 submodel, plant communities
State 1
Reference State
The Reference State 1.0 is representative of the natural range of variability under pristine conditions. The Reference State has two general community phases; a shrub-grass dominant phase and a shrub dominant phase. State dynamics are maintained by interactions between climatic patterns and disturbance regimes. Negative feedbacks enhance ecosystem resilience and contribute to the stability of the state. These include the presence of all structural and functional groups, low fine fuel loads, and retention of organic matter and nutrients. This site is very stable, with little variation in plant community composition. Plant community changes would occur in response to precipitation, long-term drought, or abusive grazing and would be reflected in annual production. Wet years will increase grass production, while normal dry conditions will reduce grass production and shrubs will dominate. Management should focus on maintaining high species diversity of desired species to promote site resiliency.
Community 1.1
Community Phase
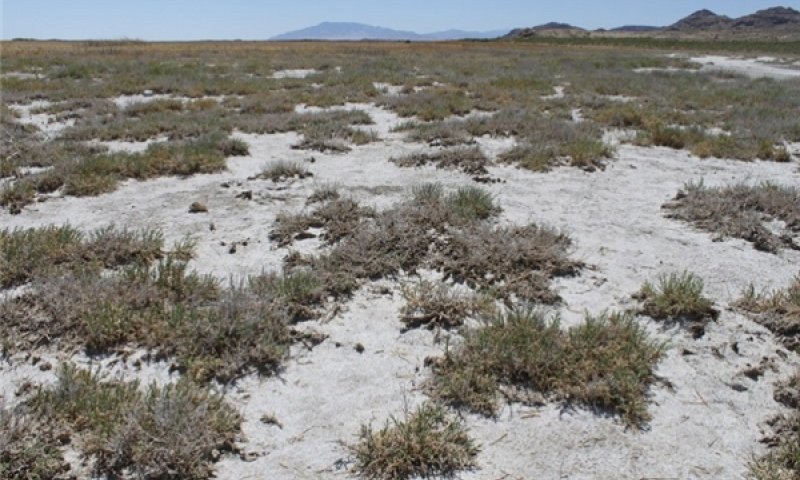
Figure 7. Saline Flat (R028AY009NV) T.Stringham May 2012
The plant community is dominated by iodinebush and inland saltgrass. Mojave seablite, black greasewood, alkali sacaton, and basin wildrye make up minor components. Potential vegetative composition is about 30% grasses, 5% forbs and 65% shrubs. Approximate ground cover (basal and crown) is 5 to 10 percent.
Figure 8. Annual production by plant type (representative values) or group (midpoint values)
Table 5. Annual production by plant type
Plant type | Low (lb/acre) |
Representative value (lb/acre) |
High (lb/acre) |
---|---|---|---|
Shrub/Vine | 49 | 65 | 98 |
Grass/Grasslike | 23 | 30 | 45 |
Forb | 3 | 5 | 7 |
Total | 75 | 100 | 150 |
Community 1.2
Community Phase
Saltgrass increases, iodinebush may decrease. Other perennial bunchgrasses such as alkali sacaton and basin wildrye may increase.
Pathway a
Community 1.1 to 1.2
Abnormally high precipitation will cause ponding on the soil surface, leading to an increase in saltgrass.
Pathway a
Community 1.2 to 1.1
Dry conditions cause salts to accumulate at the soil surface, which allows iodinebush to become dominant. Perennial bunchgrasses will be reduced.
State 2
Current Potential State
This state is similar to the Reference State 1.0 with two similar community phases. Ecological function has not changed, however the resiliency of the state has been reduced by the presence of invasive weeds. Non-natives may increase in abundance but will not become dominant within this state. These non-natives can be highly flammable and can promote fire where historically fire had been infrequent. Negative feedbacks enhance ecosystem resilience and contribute to the stability of the state. These feedbacks include the presence of all structural and functional groups, low fine fuel loads, and retention of organic matter and nutrients. Positive feedbacks reduce ecosystem resilience and stability of the state. These include the non-natives’ high seed output, persistent seed bank, rapid growth rate, ability to cross pollinate, and adaptations for seed dispersal. Management would be to maintain high diversity of desired species to promote organic matter inputs and prevent the dispersal and seed production of the non-native invasive species.
Community 2.1
Community Phase
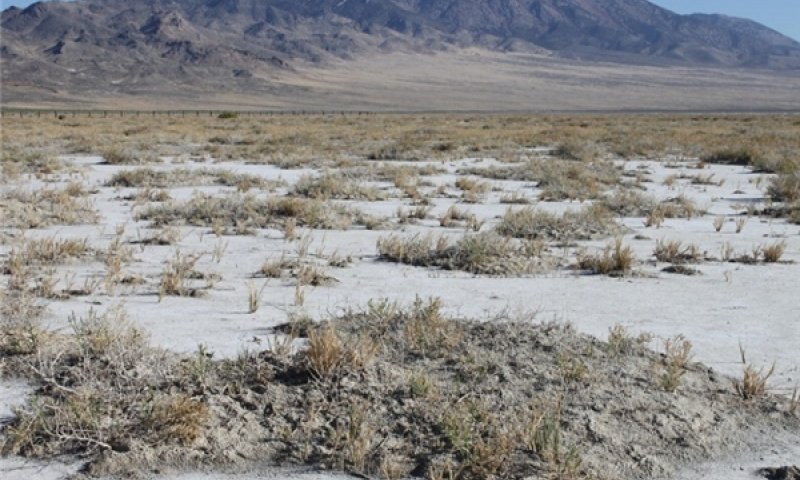
Figure 9. Saline Flat (R028AY009NV) T.Stringham May 2012
This community is dominated by iodinebush and saltgrass. Mojave seablite, black greasewood, alkali sacaton, and basin wildrye make up minor components. Annual non-native species such as halogeton and Russian thistle are present and may be increasing within the community. Saltcedar may occur in disturbed areas where water has ponded.
Community 2.2
Community Phase
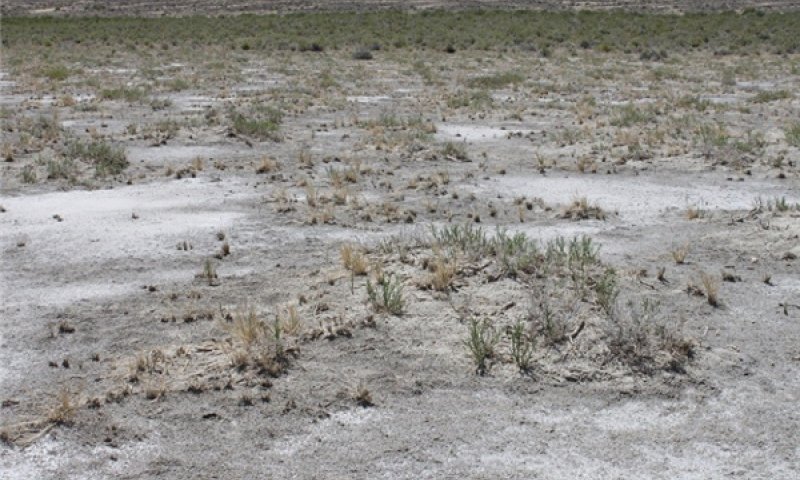
Figure 10. Saline Flat (R028AY009NV) T.Stringham May 2012
Saltgrass increases, iodinebush may decrease. Other perennial bunchgrasses such as alkali sacaton and basin wildrye may increase. Annual non-native species present and may increase with spring precipitation.
Pathway a
Community 2.1 to 2.2
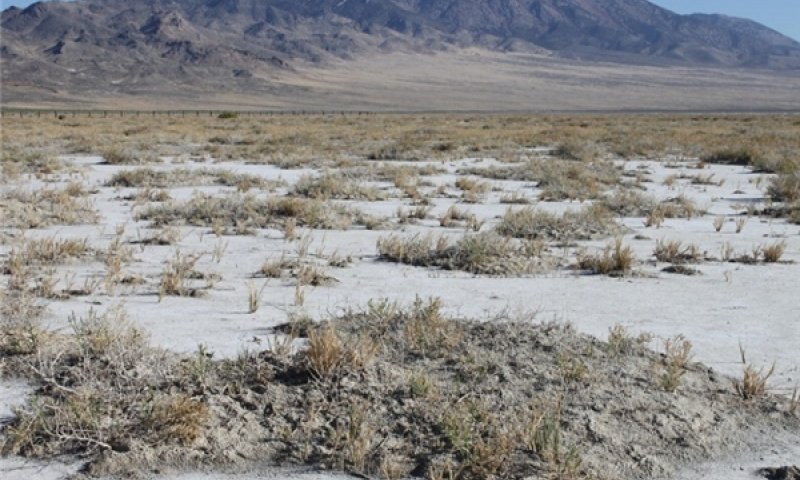
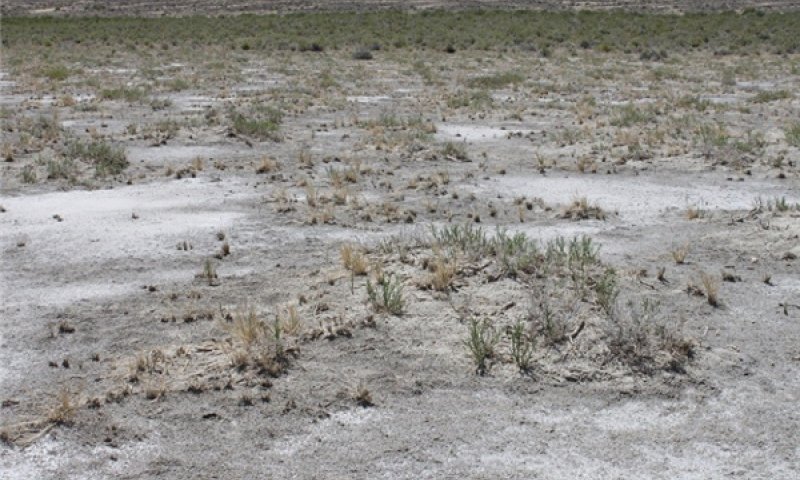
Abnormally high precipitation will cause ponding on the soil surface, leading to an increase in saltgrass.
Pathway a
Community 2.2 to 2.1
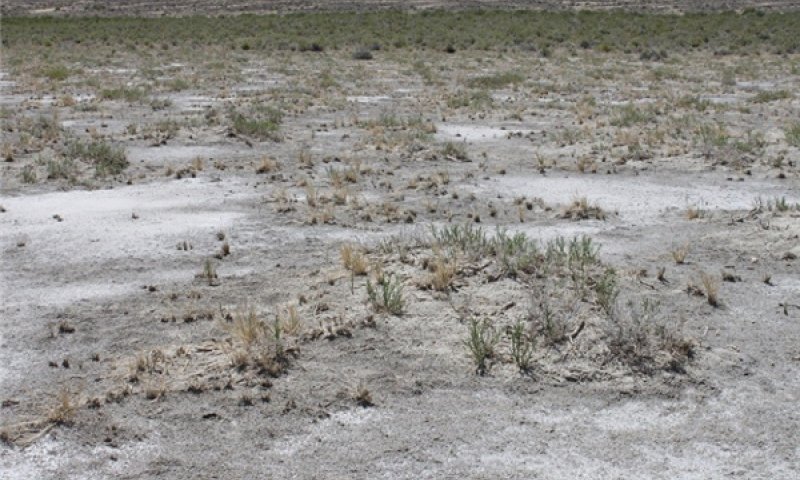
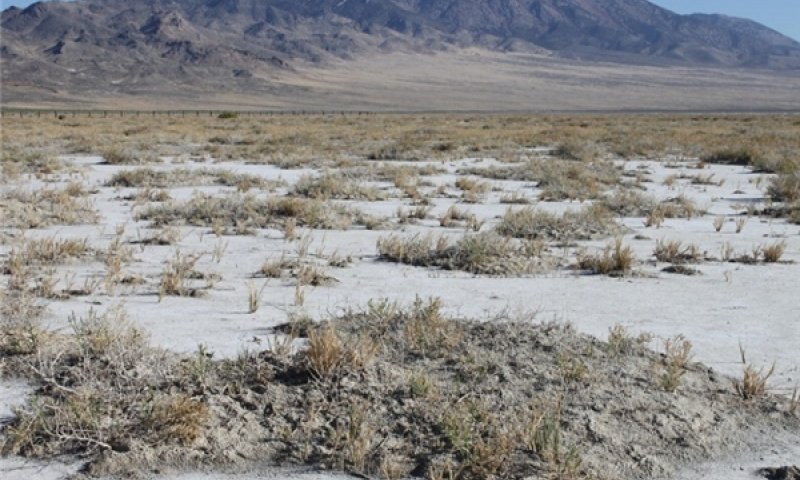
Dry conditions cause salts to accumulate at the soil surface, which allows iodinebush to become dominant. Perennial bunchgrasses will be reduced.
Transition A
State 1 to 2
Trigger: This transition is caused by the introduction of non-native annual plants such as halogeton, Russian thistle, cheatgrass, or salt cedar. Slow variables: Over time the annual non-native species will increase within the community. Threshold: The presence of introduced non-native species causes an immediate decrease in the resilience of the site. Annual non-native species cannot be easily removed from the system and have the potential to significantly alter disturbance regimes from their historic range of variation.
Additional community tables
Table 6. Community 1.1 plant community composition
Group | Common name | Symbol | Scientific name | Annual production (lb/acre) | Foliar cover (%) | |
---|---|---|---|---|---|---|
Grass/Grasslike
|
||||||
1 | Primary Perennial Grasses | 20–30 | ||||
saltgrass | DISP | Distichlis spicata | 20–30 | – | ||
2 | Secondary Perennial Grasses | 2–8 | ||||
basin wildrye | LECI4 | Leymus cinereus | 1–3 | – | ||
alkali sacaton | SPAI | Sporobolus airoides | 1–3 | – | ||
Forb
|
||||||
3 | Perennial | 2–8 | ||||
boraxweed | NIOC2 | Nitrophila occidentalis | 1–2 | 0–5 | ||
milkvetch | ASTRA | Astragalus | 1–2 | – | ||
4 | Annual | 1–2 | ||||
woollystar | ERIAS | Eriastrum | 1–2 | – | ||
Shrub/Vine
|
||||||
5 | Primary Shrubs | 50–60 | ||||
iodinebush | ALOC2 | Allenrolfea occidentalis | 50–60 | – | ||
6 | Secondary Shrubs | 16–49 | ||||
basin saltbush | ATTR3 | Atriplex tridentata | 1–3 | – | ||
spiny hopsage | GRSP | Grayia spinosa | 1–3 | – | ||
greasewood | SAVE4 | Sarcobatus vermiculatus | 1–3 | – |
Interpretations
Animal community
Livestock Interpretations:
This site has limited value for livestock grazing, due to the low forage production. Kovalev and Krylova (1992) found that iodinebush is a possible feed for animals if mixed with other forage plants. Saltgrass is an important forage species and is relatively high in crude protein (Hanson et al 1976). It is grazed by both cattle and horses and it has a forage value of fair to good because it remains green when most other grasses are dry during periods of drought as well as being resistant to grazing and trampling (Skaradek and Miller 2010, Kartesz 1988). Saltgrass's value as forage depends primarily on the relative availability of other grasses of higher nutritional value and palatability. It can be an especially important late summer grass in arid environments after other forage grasses have deceased. Saltgrass is rated as a fair to good forage species only because it stays green after most other grasses dry. Livestock generally avoid saltgrass due to its coarse foliage. Saltgrass is described as an “increaser” under grazing pressure.
Alkali sacaton provides valuable forage but has been found to be sensitive to early growing season defoliation whereas late growing season and/or dormant season use allowed recovery of depleted stands (Hickey and Springfield 1966).
Stocking rates vary over time depending upon season of use, climate variations, site, and previous and current management goals. A safe starting stocking rate is an estimated stocking rate that is fine tuned by the client by adaptive management through the year and from year to year.
Wildlife Interpretations:
Salt-desert shrub communities are relatively simple in terms of structure and species diversity but they serve as habitat for several wildlife species including reptiles, small mammals, birds and large herbivores (pronghorn) (Blaisdell and Holmgren 1984).
Iodine bush is an important forage species for many wildlife species. Saltgrass provides cover for a variety of bird species, small mammals, and arthropods and is on occasion used as forage for several big game wildlife species.
Hydrological functions
Runoff is very high. Permeability is slow.
Recreational uses
Aesthetic value is derived from the diverse floral and faunal composition and the colorful flowering of wild flowers and shrubs during the spring and early summer. This site offers rewarding opportunities to photographers and for nature study. This site is used for camping and hiking and has potential for upland and big game hunting.
Other information
Saltgrass is a suitable species for controlling wind and water erosion due to its extensive system of rhizomes and roots which form a dense sod.
Supporting information
Type locality
Location 1: Elko County, NV | |
---|---|
Township/Range/Section | T35N R70E S28 |
Latitude | 40° 53′ 13″ |
Longitude | 114° 3′ 47″ |
General legal description | NW¼NW¼, About 12 miles north of Wendover, near Little Salt Spring, Elko County, Nevada. |
Location 2: Tooele County, UT | |
Township/Range/Section | T3S R19W S32 |
Latitude | 40° 31′ 21″ |
Longitude | 114° 1′ 32″ |
General legal description | Southeast of Wendover, off Nevada Highway 24, along western margin of Great Salt Lake, Blue Lakes area, Tooele County, Utah. |
Other references
Blaisdell, J. P. and R. C. Holmgren. 1984. Managing Intermountain Rangelands - Salt-desert Shrub Ranges. General Technical Report INT-163. USDA Forest Service, Intermountain Forest and Range Experiment Station, Ogden, UT.
Blank, R.R., J.A. Young, J.D. Trent, and D.E Palmquist. 1998. Natural history of a saline mound ecosystem. Great Basin Naturalist 58:217-230.
Caudle, D., J. DiBenedetto, M. Karl, H. Sanchez, and C. Talbot. 2013. Interagency Ecological Site Handbook for Rangelands. Available at: http://jornada.nmsu.edu/sites/jornada.nmsu.edu/files/InteragencyEcolSiteHandbook.pdf. Accessed 4 October 2013.
Chambers, J., B. Bradley, C. Brown, C. D’Antonio, M. Germino, J. Grace, S. Hardegree, R. Miller, and D. Pyke. 2013. Resilience to stress and disturbance, and resistance to Bromus tectorum L. invasion in cold desert shrublands of western North America. Ecosystems:1-16.
Comstock, J. P. and J. R. Ehleringer. 1992. Plant adaptation in the Great Basin and Colorado Plateau. The Great Basin Naturalist 52(3):195-215.
de Szalay, F. A. and V. H. Resh. 1997. Responses of wetland invertebrates and plants important in waterfowl diets to burning and mowing of emergent vegetation. Wetlands 17(1):149-156.
Fire Effects Information System (Online; http://www.fs.fed.us/database/feis/plants/).
Flowers, S. 1934. Vegetation of the Great Salt Lake region. Botanical Gazette. Pp 353-418.
Gul, B. and D.J. Weber. 2001. Seed bank dynamics in a Great Basin salt playa. Journal of Arid Environments 49:785-794.
Gul, B. and D. J. Weber. 1999. Effect of salinity, light, and temperature on germination in Allenrolfea occidentalis. Canadian Journal of Botany 77(2):240-246.
Hansen, D. J.; Dayanandan, P.; Kaufman, Peter B.; Brotherson J. D. 1976. Ecological adaptations of salt marsh grass, Distichlis spicata (Gramineae), and environmental factors affecting its growth and distribution. American Journal of Botany 63(5):635-650.
Hickey, W.C. and H.W. Springfield. 1966. Alkali sacaton: Its merits for forage cover. Journal of Range Management 19:17-74.
Houghton, J.G., C.M. Sakamoto, and R.O. Gifford. 1975. Nevada’s Weather and Climate, Special Publication 2. Nevada Bureau of Mines and Geology, Mackay School of Mines, University of Nevada, Reno, NV.
Kartesz, J.T. 1988. A Flora of Nevada. University of Nevada, Reno, ProQuest, UMI Dissertations Publishing.
Kovalev, V.M. and N.P. Krylova. 1992. Use of halophytes to improve arid pastures. Sel’skokhozyaistvennaya Biologiyha 4:135-141.
Marcum, K.B. and D.H. Kopec. 1997. Salinity tolerance of turfgrasses and alternative species in the subfamily Chloridoideae (Poaceae). International Turfgrass Society Research Journal 8:735-742.
Meinzer, O.E. 1927. Plants as Indicators of Ground Water. Geological Survey Water-Supply Paper 577. U.S. Government Printing Office, Washington, D.C.
National Oceanic and Atmospheric Administration. 2004. The North American Monsoon. Reports to the Nation. National Weather Service, Climate Prediction Center. Available online: http://www.weather.gov/.
Newman, S.D. and M. Gates. 2000. Plant Guide for saltgrass Distichlis spicata (L.) Greene. USDA-Natural Resources Conservation Service National Plant Data Center & the Louisiana State Office.
Paysen, T. E., R. J. Ansley, J. K. Brown, G. J. Gottfried, S. M. Haase, M. G. Harrington, M. G. Narog, S. S. Sackett, and R. C. Wilson. 2000. Fire in western shrubland, woodland, and grassland ecosystems. Wildland Fire in Ecosystems: Effects of Fire on Flora. Gen. Tech. Rep. RMRS-GTR-42-vol 2:121-159.
Skaradek, W., and C. Miller. 2010. Saltgrass Distichlisspicata (L.) Greene. Plant Fact Sheet. U.S. Department of Agriculture, Natural Resources Conservation Service. Available from: http://plants.usda.gov/factsheet/pdf/fs_disp.pdf
Smith, L. M. and J.A. Kadlec. 1985. Comparisons of prescribed burning and cutting of Utah marsh plants. The Great Basin Naturalist 45: 462-466.
Stringham, T.K., P. Novak-Echenique, P. Blackburn, C. Coombs, D. Snyder and A. Wartgow. 2015. Final Report for USDA Ecological Site Description State-and-Transition Models, Major Land Resource Area 28A and 28B Nevada. University of Nevada Reno, Nevada Agricultural Experiment Station Research Report 2015-01. p. 1524.
Trent, J. D., R. R. Blank, and J. A. Young. 1997. Ecophysiology of the temperate desert halophytes: Allenrolfea occidentalis and Sarcobatus vermiculatus. Western North American Naturalist 57(1), 57-65.
Ungar, I. A. 1982. Germination ecology of halophytes. In Contributions to the Ecology of Halophytes Springer Netherlands. Pp. 143-154.
USDA, Forest Service. 1988. Range Plant Handbook. Dover Publications, Inc. New York, NY.
USDA, NRCS. 2015. The PLANTS Database (http://plants.usda.gov, 5 January 2015). National Plant Data Team, Greensboro, NC.
Weber, D., B. Gul, and M. A. Khan. 1998. Population dynamics of a perennial halophyte Allenrolfea ocidentalis. In: E.D. McArthur, W. K. Ostler, C.L. Wambolt, comps. 1999. Proceedings: shrubland ecotones; 1998 August 12-14; Ephraim, UT. Proc. RMRS-P-11. Ogden, UT: U.S. Department of Agriculture, Forest Service, Rocky Mountain Research Station. 299 p.
Weber, D., B. Gul, and M. A. Khan. 2002. Halophytic characteristics and potential uses of Allenrolfea occidentalis. In: R. Ahmad and K. A. Malik, editors. Prospects for Saline Agriculture. Springer Netherlands. p 333-352.
West, N. E. (1994). Effects of fire on salt-desert shrub rangelands. In: Proceedings: Ecology and management of annual rangelands. Ogden, UT: US Department of Agriculture, Forest Service, Intermountain Research Station. Gen. Tech. Rep. INT-313. P 71-74.
Young, R.P. 1983. Fire as a vegetation management tool in rangelands of the Intermountain Region. In: Monsen, S.B. and N. Shaw (compilers). Managing Intermountain rangelands-- improvement of range and wildlife habitats: Proceedings; 1981 September 15-17; Twin Falls, ID; 1982 June 22-24; Elko, NV. Ogden, UT: U.S. Department of Agriculture, Forest Service, Intermountain Forest and Range Experiment Station. Gen. Tech. Rep. INT-157. p 18-31.
Contributors
RK
T. Stringham/P.Novak-Echenique
Rangeland health reference sheet
Interpreting Indicators of Rangeland Health is a qualitative assessment protocol used to determine ecosystem condition based on benchmark characteristics described in the Reference Sheet. A suite of 17 (or more) indicators are typically considered in an assessment. The ecological site(s) representative of an assessment location must be known prior to applying the protocol and must be verified based on soils and climate. Current plant community cannot be used to identify the ecological site.
Author(s)/participant(s) | P NOVAK-ECHENIQUE |
---|---|
Contact for lead author | State Rangeland Management Specialist |
Date | 05/20/2013 |
Approved by | |
Approval date | |
Composition (Indicators 10 and 12) based on | Annual Production |
Indicators
-
Number and extent of rills:
This site is essentially level and rills do not form. -
Presence of water flow patterns:
Water flow patterns are rare to common depending on site location relative to major inflow areas. Flow patterns may be (up to 15 ft) and widely spaced (>10 ft apart). They are stable with only minor evidence of deposition. -
Number and height of erosional pedestals or terracettes:
Plants may have small pedestals where they are adjacent to water flow paths. There are no terracettes. -
Bare ground from Ecological Site Description or other studies (rock, litter, lichen, moss, plant canopy are not bare ground):
Bare Ground ± 80%. -
Number of gullies and erosion associated with gullies:
None -
Extent of wind scoured, blowouts and/or depositional areas:
Small depositional areas occur creating small mounds at the base of shrubs. -
Amount of litter movement (describe size and distance expected to travel):
Fine litter (foliage of grasses and annual & perennial forbs) expected to move distance of slope length during periods of intense summer convection storms or run in of early spring snow melt flows. Persistent litter (large woody material) will remain in place except during unusual flooding (ponding) events. -
Soil surface (top few mm) resistance to erosion (stability values are averages - most sites will show a range of values):
Soil stability values will range from 2 to 4. (To be field tested.) -
Soil surface structure and SOM content (include type of structure and A-horizon color and thickness):
Structure of soil surface is weak platy. Soil surface colors are grays and soils are typified by an ochric epipedon. Surface textures are silt loams. Organic matter is typically less than 1 percent. -
Effect of community phase composition (relative proportion of different functional groups) and spatial distribution on infiltration and runoff:
Sparse cover of shrubs and rhizomatous grasses slow runoff and aid in infiltration. Moderately fine to fine surface textures and physical crusts result in limited infiltration rates. The surface layer will normally crust and bake upon drying, inhibiting water infiltration and seedling emergence. -
Presence and thickness of compaction layer (usually none; describe soil profile features which may be mistaken for compaction on this site):
Compacted layers are none. Subsurface massive structure is normal for this site and should not to be interpreted as compaction. -
Functional/Structural Groups (list in order of descending dominance by above-ground annual-production or live foliar cover using symbols: >>, >, = to indicate much greater than, greater than, and equal to):
Dominant:
Reference State: salt-desert shrubs (iodinebush)Sub-dominant:
warm season, rhizomatous grasses > associated shrubs > deep-rooted perennial bunchgrasses = deep-rooted, cool season, perennial forbs > fibrous, shallow-rooted, cool season, perennial and annual forbs.Other:
microbiotic crustsAdditional:
-
Amount of plant mortality and decadence (include which functional groups are expected to show mortality or decadence):
Dead branches within individual shrubs common and standing dead shrub canopy material may be as much as 35% of total woody canopy. -
Average percent litter cover (%) and depth ( in):
Between plant interspaces (5-10%) and depth (< ¼ in.) -
Expected annual annual-production (this is TOTAL above-ground annual-production, not just forage annual-production):
For normal or average growing season (thru July) ± 100 lbs/ac. Favorable years ± 150 lbs/ac and unfavorable years ± 75 lbs/ac. -
Potential invasive (including noxious) species (native and non-native). List species which BOTH characterize degraded states and have the potential to become a dominant or co-dominant species on the ecological site if their future establishment and growth is not actively controlled by management interventions. Species that become dominant for only one to several years (e.g., short-term response to drought or wildfire) are not invasive plants. Note that unlike other indicators, we are describing what is NOT expected in the reference state for the ecological site:
Potential invaders include salt cedar, annual mustards, Russian thistle, and halogeton. -
Perennial plant reproductive capability:
All functional groups should reproduce in average (or normal) and above average growing season years. Reduced growth and reproduction occurs during extreme or extended drought conditions.
Print Options
Sections
Font
Other
The Ecosystem Dynamics Interpretive Tool is an information system framework developed by the USDA-ARS Jornada Experimental Range, USDA Natural Resources Conservation Service, and New Mexico State University.
Click on box and path labels to scroll to the respective text.