
Natural Resources
Conservation Service
Ecological site R110XY009IL
Dry Glacial Drift Upland Savanna
Last updated: 4/22/2020
Accessed: 07/17/2024
General information
Provisional. A provisional ecological site description has undergone quality control and quality assurance review. It contains a working state and transition model and enough information to identify the ecological site.
MLRA notes
Major Land Resource Area (MLRA): 110X–Northern Illinois and Indiana Heavy Till Plain
The Northern Illinois and Indiana Heavy Till Plain (MLRA 110) encompasses the Northeastern Morainal, Grand Prairie, and Southern Lake Michigan Coastal landscapes (Schwegman et al. 1973, WDNR 2015). It spans three states – Illinois (79 percent), Indiana (10 percent), and Wisconsin (11 percent) – comprising about 7,535 square miles (Figure 1). The elevation is about 650 feet above sea level (ASL) and increases gradually from Lake Michigan south. Local relief varies from 10 to 25 feet. Silurian age fractured dolomite and limestone bedrock underlie the region. Glacial drift covers the surface area of the MLRA, and till, outwash, lacustrine deposits, loess or other silty material, and organic deposits are common (USDA-NRCS 2006).
The vegetation in the MLRA has undergone drastic changes over time. At the end of the last glacial episode – the Wisconsinan glaciation – the evolution of vegetation began with the development of tundra habitats, followed by a phase of spruce and fir forests, and eventually spruce-pine forests. Not until approximately 9,000 years ago did the climate undergo a warming trend which prompted the development of deciduous forests dominated by oak and hickory. As the climate continued to warm and dry, prairies began to develop approximately 8,300 years ago. Another shift in climate that resulted in an increase in moisture prompted the emergence of savanna-like habitats from 8,000 to 5,000 years before present (Taft et al. 2009). Forests maintained footholds on steep valley sides, morainal ridges, and wet floodplains. Fire, droughts, and grazing by native mammals helped to maintain the prairies and savannas until the arrival of European settlers, and the forests were maintained by droughts, wind, lightning, and occasional fire (Taft et al. 2009; NatureServe 2018).
Classification relationships
USFS Subregions: Southwestern Great Lakes Morainal (222K) and Central Till Plains and Grand Prairies (251D) Sections; Kenosha-Lake Michigan Plain and Moraines (222Kg), Valparaiso Moraine (Kj), and Eastern Grand Prairie (251Dd) Subsections (Cleland et al. 2007)
U.S. EPA Level IV Ecoregion: Kettle Moraines (53b), Illinois/Indiana Prairies (54a), and Valparaiso-Wheaton Morainal Complex (54f) (USEPA 2013)
National Vegetation Classification – Ecological Systems: North-Central Interior Oak Savanna (CES202.698) (NatureServe 2018)
National Vegetation Classification – Plant Associations: Quercus macrocarpa – (Quercus alba, Quercus velutina)/ Andropogon gerardii Wooded Grassland (CEGL002020) (Nature Serve 2018)
Biophysical Settings: North-Central Interior Oak Savanna (BpS 4213940) (LANDFIRE 2009)
Illinois Natural Areas Inventory: Dry-mesic savanna (White and Madany 1978)
Wisconsin Natural Communities: Oak Opening (WDNR 2015)
Ecological site concept
Dry Glacial Drift Upland Savannas are located within the green areas on the map. They occur on uplands. The soils are Mollisols and Alfisols that are well drained and very deep, formed in loess, loamy outwash, glacial till, lacustrine deposits, or glaciofluvial deposits.
The historic pre-European settlement vegetation on this ecological site was dominated by tallgrass prairie interspersed with trees. Bur oak (Quercus macrocarpa Michx.) is the dominant tree, and little bluestem (Schizachyrium scoparium (Michx.) Nash) and porcupinegrass (Hesperostipa spartea (Trin.) Barkworth) are the dominant grasses on the site. Other grasses present can include Indiangrass (Sorghastrum nutans (L.) Nash) and big bluestem (Andropogon gerardii Vitman) (White and Madany 1978; NatureServe 2018). Forbs typically associated with an undisturbed plant community associated with this ecological site can include wild quinine (Parthenium integrifolium L.) (White and Madany 1978; Taft et al. 1997). Fire is the primary disturbance factor that maintains this site, while herbivory and drought are secondary factors (LANDFIRE 2009).
Associated sites
R110XY010IL |
Moist Glacial Drift Upland Savanna Loess or other silty or loamy material, loamy outwash, glacial till, or lacustrine deposits parent material that has a seasonal high-water table within 12-72 inches including Beardstown, Beecher, Chatsworth, Frankfort, Graymont, Grays, Markham, Millbrook, and Wauconda soils |
---|
Table 1. Dominant plant species
Tree |
(1) Quercus macrocarpa |
---|---|
Shrub |
Not specified |
Herbaceous |
(1) Schizachyrium scoparium |
Physiographic features
Dry Glacial Drift Upland Savannas occur on uplands. They are situated on elevations ranging from approximately 512 to 1148 feet ASL. The site does not experience flooding but rather generates runoff to adjacent, downslope ecological sites.
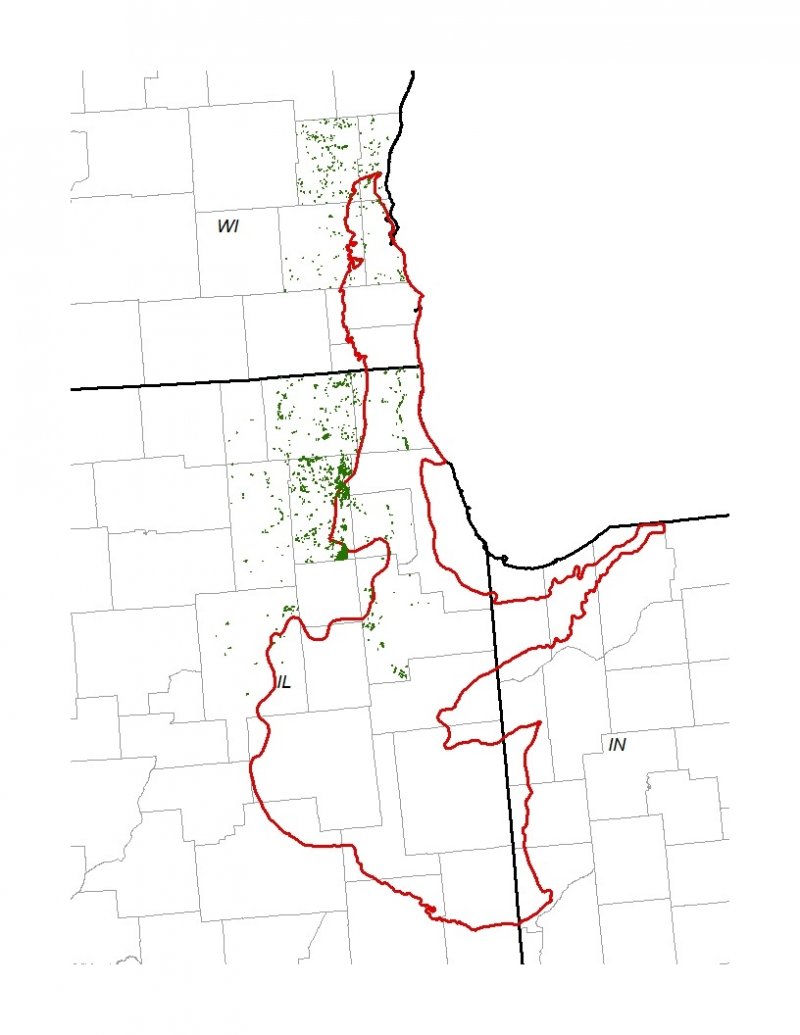
Figure 1.
Table 2. Representative physiographic features
Slope shape across |
(1) Convex |
---|---|
Slope shape up-down |
(1) Convex |
Landforms |
(1)
Upland
|
Runoff class | Low to high |
Elevation | 512 – 1,148 ft |
Slope | 2 – 10% |
Water table depth | 80 in |
Aspect | Aspect is not a significant factor |
Climatic features
The Northern Illinois and Indiana Heavy Till Plain falls into the hot-summer humid continental climate (Dfa) and warm-summer humid continental climate (Dfb) Köppen-Geiger climate classifications (Peel et al. 2007). The two main factors that drive the climate of the MLRA are latitude and weather systems. Latitude, and the subsequent reflection of solar input, determines air temperatures and seasonal variations. Solar energy varies across the seasons, with summer receiving three to four times as much energy as opposed to winter. Weather systems (air masses and cyclonic storms) are responsible for daily fluctuations of weather conditions. High-pressure systems are responsible for settled weather patterns where sun and clear skies dominate. In fall, winter, and spring, the polar jet stream is responsible for the creation and movement of low-pressure systems. The clouds, winds, and precipitation associated with a low-pressure system regularly follow high-pressure systems every few days (Angel n.d.).
The soil temperature regime of MLRA 110 is classified as mesic, where the mean annual soil temperature is between 46 and 59°F (USDA-NRCS 2006). Temperature and precipitation occur along a north-south gradient, where temperature and precipitation increase the further south one travels. The average freeze-free period of this ecological site is about 165 days, while the frost-free period is about 134 days (Table 2). The majority of the precipitation occurs as rainfall in the form of convective thunderstorms during the growing season. Average annual precipitation is 37 inches, which includes rainfall plus the water equivalent from snowfall (Table 3). The average annual low and high temperatures are 39.1 and 58.6°F, respectively.
Table 3. Representative climatic features
Frost-free period (characteristic range) | 125-144 days |
---|---|
Freeze-free period (characteristic range) | 150-182 days |
Precipitation total (characteristic range) | 36-37 in |
Frost-free period (actual range) | 115-151 days |
Freeze-free period (actual range) | 131-192 days |
Precipitation total (actual range) | 35-38 in |
Frost-free period (average) | 134 days |
Freeze-free period (average) | 165 days |
Precipitation total (average) | 37 in |
Figure 2. Monthly precipitation range
Figure 3. Monthly minimum temperature range
Figure 4. Monthly maximum temperature range
Figure 5. Monthly average minimum and maximum temperature
Figure 6. Annual precipitation pattern
Figure 7. Annual average temperature pattern
Climate stations used
-
(1) GIBSON CITY [USC00113413], Gibson City, IL
-
(2) JOLIET BRANDON RD DAM [USC00114530], Joliet, IL
-
(3) ELGIN [USC00112736], Elgin, IL
-
(4) GERMANTOWN [USC00473058], Germantown, WI
Influencing water features
Dry Glacial Drift Upland Savannas are not influenced by wetland or riparian water features. Precipitation is the main source of water for this ecological site. Infiltration is moderate (Hydrologic Group B), and surface runoff is low to high. Surface runoff contributes some water to downslope ecological sites.
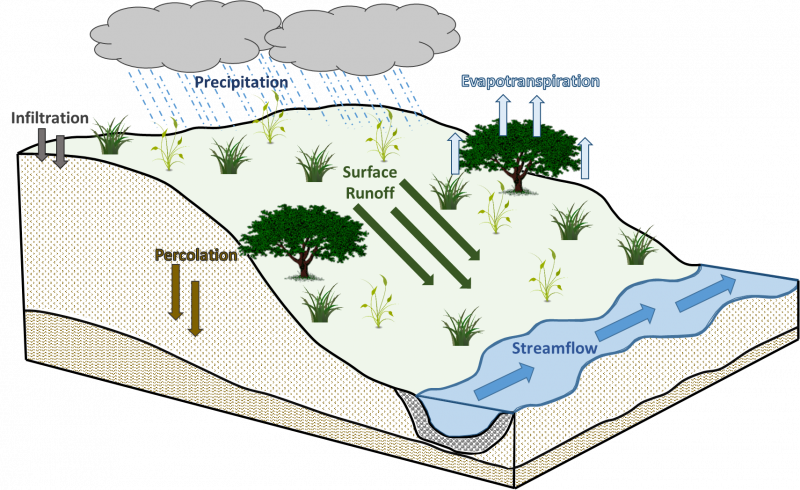
Figure 8. Hydrologic cycling in Dry Glacial Drift Upland Savanna ecological site.
Soil features
Soils of Dry Glacial Drift Upland Savannas are in the Mollisols and Alfisols orders, further classified as Mollic Hapludalfs, and Mollic Oxyaquic Hapludalfs with moderate infiltration and low to high runoff potential. The soil series associated with this site includes Bowes, Dresden, and Grays. The parent material is loess or other silty or loamy material, loamy outwash, glacial till, lacustrine deposits, or glaciofluvial deposits and the soils are well drained and very deep. Soil pH classes are very strongly acid to moderately alkaline. No rooting restrictions are noted for the soils of this ecological site.
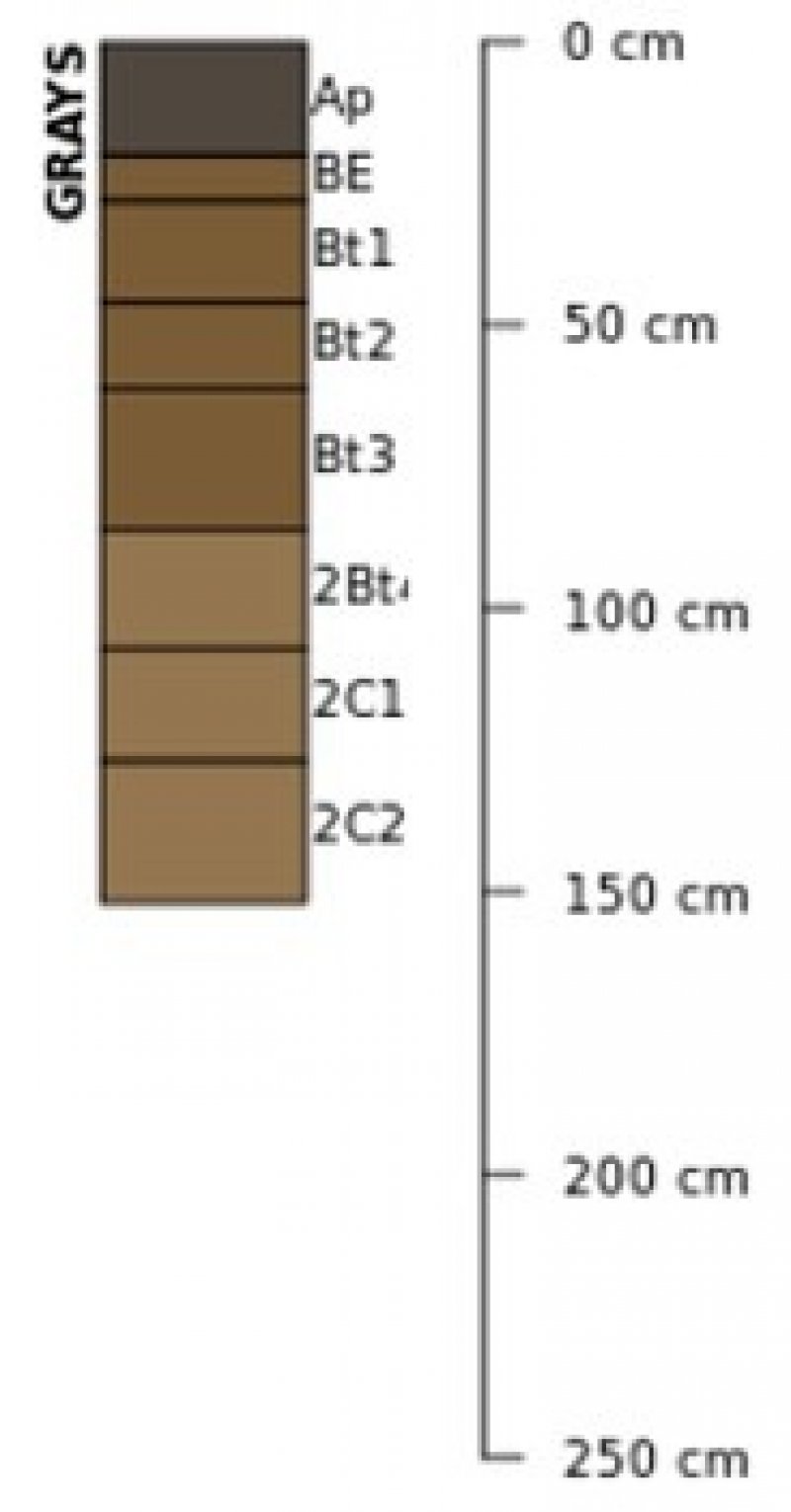
Figure 9. Profile sketches of soil series associated with Dry Glacial Drift Upland Savanna.
Table 4. Representative soil features
Parent material |
(1)
Loess
(2) Outwash (3) Till (4) Lacustrine deposits (5) Glaciofluvial deposits |
---|---|
Family particle size |
(1) Fine-silty (2) Fine-loamy (3) Fine-loamy over sandy or sandy-skeletal |
Drainage class | Well drained |
Permeability class | Slow to moderately slow |
Depth to restrictive layer | 80 in |
Soil depth | 80 in |
Surface fragment cover <=3" | Not specified |
Surface fragment cover >3" | Not specified |
Available water capacity (Depth not specified) |
5 – 8 in |
Calcium carbonate equivalent (Depth not specified) |
40% |
Electrical conductivity (Depth not specified) |
2 mmhos/cm |
Sodium adsorption ratio (Depth not specified) |
Not specified |
Soil reaction (1:1 water) (Depth not specified) |
4.5 – 8.4 |
Subsurface fragment volume <=3" (Depth not specified) |
2 – 32% |
Subsurface fragment volume >3" (Depth not specified) |
1 – 19% |
Ecological dynamics
The information in this Ecological Site Description, including the state-and-transition model (STM), was developed based on historical data, current field data, professional experience, and a review of the scientific literature. As a result, all possible scenarios or plant species may not be included. Key indicator plant species, disturbances, and ecological processes are described to inform land management decisions.
The MLRA lies within the tallgrass prairie ecosystem of the Midwest, but a variety of environmental and edaphic factors resulted in landscape that historically supported prairies, savannas, forests, and various wetlands. Dry Glacial Drift Upland Savannas form an aspect of this vegetative continuum. This ecological site occurs on uplands on well drained soils. Species characteristic of this ecological site consist of herbaceous vegetation with scattered trees.
Fire is a critical disturbance factor that maintains Dry Glacial Drift Upland Savannas. Fire intensity typically consisted of periodic fires occurring every 1 to 5 years (LANDFIRE 2009). Ignition sources included summertime lightning strikes from convective storms and bimodal, human ignitions during the spring and fall seasons. Native Americans regularly set fires to improve sight lines for hunting, driving large game, improving grazing and browsing habitat, agricultural clearing, and enhancing vital ethnobotanical plants (Barrett 1980; White 1994).
Drought and herbivory by native ungulates have also played a role in shaping this ecological site. The periodic episodes of reduced soil moisture in conjunction with the well drained soils have favored the proliferation of plant species tolerant of such conditions. Drought can also slow the growth of plants and result in dieback of certain species. Bison (Bos bison) grazing, while present, served a more limited role in community composition and structure than lands further west. Prairie elk (Cervus elaphus) and white-tailed deer (Odocoileus virginianus) likely contributed to woody species reduction but are also considered to be of a lesser impact compared to the west (LANDFIRE 2009). When coupled with fire, periods of drought and herbivory can further delay the establishment of woody vegetation (Pyne et al. 1996).
Today, Dry Glacial Drift Upland Savannas are virtually extinct, having been type-converted to agricultural production land or other human-modified landscapes. Remnants that do exist show evidence of indirect anthropogenic influences from fire suppression and non-native species invasion. A return to the historic plant community may not be possible following extensive land modification, but long-term conservation agriculture or prairie reconstruction efforts can help to restore some biotic diversity and ecological function. The state-and-transition model that follows provides a detailed description of each state, community phase, pathway, and transition. This model is based on available experimental research, field observations, literature reviews, professional consensus, and interpretations.
State and transition model
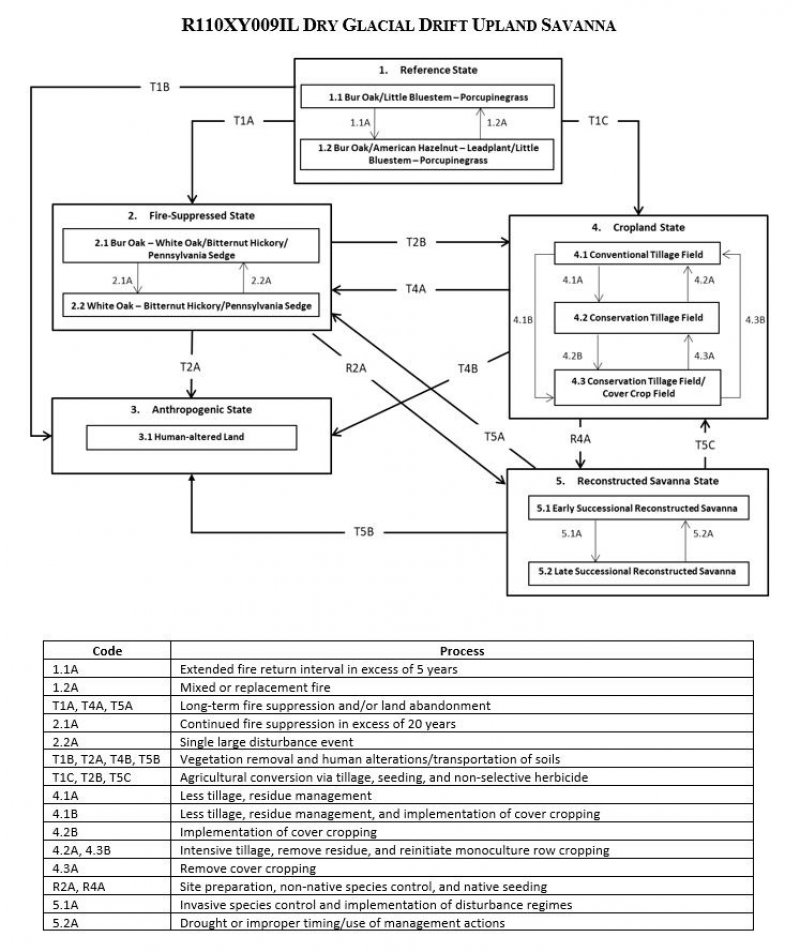
More interactive model formats are also available.
View Interactive Models
More interactive model formats are also available.
View Interactive Models
Click on state and transition labels to scroll to the respective text
Ecosystem states
States 2 and 5 (additional transitions)
State 1 submodel, plant communities
State 2 submodel, plant communities
State 3 submodel, plant communities
State 4 submodel, plant communities
State 5 submodel, plant communities
State 1
Reference State
The reference plant community is categorized as a mesic oak savanna, dominated by herbaceous vegetation and scattered trees. The two community phases within the reference state are dependent on periodic fires. Surface fires are the dominant fire regime, comprising approximately 96 percent of all fires and occurring every five years. Mixed and replacement fires comprise the remaining 4 percent, occurring approximately every 3 and 1 years, respectively (LANDFIRE 2009). Fire intensity and return intervals alter species composition, cover, and extent, while regular fire intervals keep woody species from encroaching. Episodic droughts and storm damage have more localized impacts in the reference phases, but do contribute to overall species composition, diversity, cover, and productivity.
Community 1.1
Bur Oak/Little Bluestem - Porcupinegrass
Sites in this reference community phase are dominated by a mix of grasses and forbs with scattered trees. Bur oak is the dominant tree on the site, but white oak (Quercus alba L.) may also be present. The tree layer comprises no more than 20 percent cover and tree size class is medium (9 to 21-inch DBH). Vegetative cover is continuous (up to 100 percent) and plants can reach heights up to 3 feet tall (LANDFIRE 2009). Big bluestem, little bluestem, Indiangrass, and porcupinegrass are the dominant warm-season grasses present on the site. Characteristic forbs can include wild quinine, windowsfrill (Silene stellata (l.) W.T. Aiton), and Blue Ridge carrionflower (Smilax lasioneura Hook.) (White and Madany 1978). Surface fires every 5 years will maintain this class, but an extended fire return interval will shift the community to phase 1.2 (LANDFIRE 2009).
Dominant plant species
-
bur oak (Quercus macrocarpa), tree
-
little bluestem (Schizachyrium scoparium), grass
-
porcupinegrass (Hesperostipa spartea), grass
Community 1.2
Bur Oak/American Hazelnut - Leadplant/Little Bluestem - Porcupinegrass
This reference community phase represents a successional shift as a result of an extended fire return interval. This fire-free period allows woody shrubs to establish, including American hazelnut (Corylus americana Walter) and leadplant (Amorpha canescens Pursh). Tree cover increases to as much as 60 percent, and tree size class moves from medium to large (21 to 33-inch DBH). Surface fires every 5 years will maintain this class, but mixed or replacement fires will shift the community back to phase 1.1 (LANDFIRE 2009).
Dominant plant species
-
bur oak (Quercus macrocarpa), tree
-
American hazelnut (Corylus americana), shrub
-
leadplant (Amorpha canescens), shrub
-
little bluestem (Schizachyrium scoparium), grass
-
porcupinegrass (Hesperostipa spartea), grass
Pathway 1.1A
Community 1.1 to 1.2
Extended fire return interval in excess of 5 years.
Pathway 1.2A
Community 1.2 to 1.1
Mixed or replacement fire.
State 2
Fire-Suppressed State
Fire suppression can transition the reference plant community from an open savanna to a closed canopy forest. As the natural fire regime is removed from the landscape, encroachment and dominance by shade-tolerant, fire-intolerant species ensues (Asbjornsen et al. 2005). This results in a positive feedback loop of mesophication whereby plant community succession continuously creates cool, damp shaded conditions that perpetuate a closed canopy ecosystem (Nowacki and Abrams 2008). Succession to this closed canopy state can occur in as little as 25 years from the last fire (LANDFIRE 2009).
Community 2.1
Bur Oak - White Oak/Bitternut Hickory/Pennsylvania Sedge
This community represents the early stages of long-term fire suppression. The lack of fire allows the tree canopy to close (60 to 80 percent cover) and stem density to increase. Bur oak and white oak are the dominant trees, and bitternut hickory (Carya cordiformis (Wangenh.) K. Koch) begins to form a scattered subcanopy (LANDFIRE 2009). The sun-loving herbaceous layer is slowly replaced by more shade-tolerant species. Rare surface fires that escape suppression efforts will maintain this phase, but a complete lack of fire for 30 years will shift the community to phase 2.2 (LANDFIRE 2009).
Dominant plant species
-
bur oak (Quercus macrocarpa), tree
-
white oak (Quercus alba), tree
-
bitternut hickory (Carya cordiformis), shrub
-
Pennsylvania sedge (Carex pensylvanica), other herbaceous
Community 2.2
White Oak - Bitternut Hickory/Pennsylvania Sedge
Sites falling into this community phase have transitioned into a closed canopy oak-hickory forest. Mature bur and white oaks are still present, but seedings and saplings are generally absent as they do not establish well under the continuous canopies (Tirmenstein 1991; Gucker 2011). As fire suppression has continued, bitternut hickory saplings have matured and now become co-dominant with the oaks (Colandonato 1992; LANDFIRE 2009). The ground layer continues to be dominated by shade-tolerant species or non-native invasive species, such as garlic mustard (Alliaria petiolata (M. Bieb.) Cavara & Grande). A single, large disturbance event – such as a windstorm – can create open pockets that allow the community to temporarily shift back to phase 2.1.
Dominant plant species
-
white oak (Quercus alba), tree
-
bitternut hickory (Carya cordiformis), tree
-
Pennsylvania sedge (Carex pensylvanica), other herbaceous
Pathway 2.1A
Community 2.1 to 2.2
Continued fire suppression (30+ years).
Pathway 2.2A
Community 2.2 to 2.1
Single large disturbance event.
State 3
Anthropogenic State
The anthropogenic state occurs when the reference state is cleared and developed for human use and inhabitation, such as for commercial and housing developments, landfills, parks, golf courses, cemeteries, earthen spoils, etc. The native vegetation has been removed and soils have either been altered in place (e.g. cemeteries) or transported from one location to another (e.g. housing developments). Most of the soils in this state have 50 to 100 cm of overburden on top of the natural soil. This natural material can be determined by observing a buried surface horizon or the unaltered subsoil, till, or lacustrine parent materials. This state is generally considered permanent.
Community 3.1
Human-altered land
Sites in this community phase have had the native plant community removed and soils heavily re-worked in support of human development projects.
State 4
Cropland State
The continuous use of tillage, row-crop planting, and chemicals (i.e., herbicides, fertilizers, etc.) has effectively eliminated the reference community and many of its natural ecological functions in favor of crop production. Corn and soybeans are the dominant crops for the site, and common wheat (Triticum aestivum L.) and alfalfa (Medicago sativa L.) may be rotated periodically. These areas are likely to remain in crop production for the foreseeable future.
Community 4.1
Conventional Tillage Field
Sites in this community phase typically consist of monoculture row-cropping maintained by conventional tillage practices. They are cropped in either continuous corn or corn-soybean rotations. The frequent use of deep tillage, low crop diversity, and bare soil conditions during the non-growing season negatively impacts soil health. Under these practices, soil aggregation is reduced or destroyed, soil organic matter is reduced, erosion and runoff are increased, and infiltration is decreased, which can ultimately lead to undesirable changes in the hydrology of the watershed (Tomer et al. 2005).
Community 4.2
Conservation Tillage Field
This community phase is characterized by rotational crop production that utilizes various conservation tillage methods to promote soil health and reduce erosion. Conservation tillage methods include strip-till, ridge-till, vertical-till, or no-till planting systems. Strip-till keeps seedbed preparation to narrow bands less than one-third the width of the row where crop residue and soil consolidation are left undisturbed in-between seedbed areas. Strip-till planting may be completed in the fall and nutrient application either occurs simultaneously or at the time of planting. Ridge-till uses specialized equipment to create ridges in the seedbed and vegetative residue is left on the surface in between the ridges. Weeds are controlled with herbicides and/or cultivation, seedbed ridges are rebuilt during cultivation, and soils are left undisturbed from harvest to planting. Vertical-till systems employ machinery that lightly tills the soil and cuts up crop residue, mixing some of the residue into the top few inches of the soil while leaving a large portion on the surface. No-till management is the most conservative, disturbing soils only at the time of planting and fertilizer application. Compared to conventional tillage systems, conservation tillage methods can improve soil ecosystem function by reducing soil erosion, increasing organic matter and water availability, improving water quality, and reducing soil compaction.
Community 4.3
Conservation Tillage Field/Alternative Crop Field
This community phase applies conservation tillage methods as described above as well as adds cover crop practices. Cover crops typically include nitrogen-fixing species (e.g., legumes), small grains (e.g., rye, wheat, oats), or forage covers (e.g., turnips, radishes, rapeseed). The addition of cover crops not only adds plant diversity but also promotes soil health by reducing soil erosion, limiting nitrogen leaching, suppressing weeds, increasing soil organic matter, and improving the overall soil ecosystem. In the case of small grain cover crops, surface cover and water infiltration are increased, while forage covers can be used to graze livestock or support local wildlife. Of the three community phases for this state, this phase promotes the greatest soil sustainability and improves ecological functioning within a cropland system.
Pathway 4.1A
Community 4.1 to 4.2
Tillage operations are greatly reduced, crop rotation occurs on a regular interval, and crop residue remains on the soil surface.
Pathway 4.1B
Community 4.1 to 4.3
Tillage operations are greatly reduced or eliminated, crop rotation occurs on a regular interval, crop residue remains on the soil surface, and cover crops are planted following crop harvest.
Pathway 4.2A
Community 4.2 to 4.1
Intensive tillage is utilized, and monoculture row-cropping is established.
Pathway 4.2B
Community 4.2 to 4.3
Cover crops are implemented to minimize soil erosion.
Pathway 4.3B
Community 4.3 to 4.1
Intensive tillage is utilized, cover crop practices are abandoned, monoculture row-cropping is established, and crop rotation is reduced or eliminated.
Pathway 4.3A
Community 4.3 to 4.2
Cover crop practices are abandoned.
State 5
Reconstructed Savanna State
Savanna reconstructions have become an important tool for repairing natural ecological functions and providing habitat protection for numerous grassland dependent species. Because the historic plant and soil biota communities of the tallgrass prairie were highly diverse with complex interrelationships, historic savanna replication cannot be guaranteed on landscapes that have been so extensively manipulated for extended timeframes (Kardol and Wardle 2010; Fierer et al. 2013). Therefore, ecological restoration should aim to aid the recovery of degraded, damaged, or destroyed ecosystems. A successful restoration will have the ability to structurally and functionally sustain itself, demonstrate resilience to the natural ranges of stress and disturbance, and create and maintain positive biotic and abiotic interactions (SER 2002). The reconstructed savanna state is the result of a long-term commitment involving a multi-step, adaptive management process. Bur oak plantings or selective tree thinning of non-oak species will be required to reproduce the overstory canopy (Asbjornsen et al. 2005). Diverse, species-rich seed mixes may be important to utilize as they allow the site to undergo successional stages that exhibit changing composition and dominance over time (Smith et al. 2010). On-going management via prescribed fire and/or light grazing will help the site progress from an early successional community dominated by annuals and some weeds to a later seral stage composed of native perennial grasses, forbs, shrubs, and eventually mature bur oaks. Establishing a prescribed fire regime that mimics natural disturbance patterns can increase native species cover and diversity while reducing cover of non-native forbs and grasses. Light grazing alone can help promote species richness, while grazing accompanied with fire can control the encroachment of undesirable woody vegetation (Brudvig et al. 2007).
Community 5.1
Early Successional Reconstructed Savanna
This community phase represents early community assembly and is highly dependent on the timing and priority of planting and/or tree thinning operations and the herbaceous seed mix utilized. If bur oak planting is needed, acorns should be planted shortly after harvest as acorns germinate shortly after seedfall and require no cold stratification. Browse protection may need to be installed to protect newly established seedlings from animal predation (Gucker 2011). If selective tree removal is needed, canopy reduction should encompass between 16 to 45 percent of the undesirable species in a single year (Asbjornsen et al. 2005). The seed mix should look to include a diverse mix of native cool-season and warm-season annual and perennial grasses and forbs typical of the reference state. Native, cool-season annuals can help to provide litter that promotes cool, moist soil conditions to the benefit of the other species in the seed mix. The first season following site preparation and seeding will typically result in annuals and other volunteer species forming most the vegetative cover. Control of non-native species, particularly perennial species, is crucial at this point to ensure they do not establish before the native vegetation (Martin and Wilsey 2012). After the first season, native warm-season grasses should begin to become more prominent on the landscape and over time close the canopy.
Community 5.2
Late Successional Reconstructed Savanna
Appropriately timed disturbance regimes (e.g., prescribed fire) applied to the early successional community phase can help increase the beta diversity, pushing the site into a late successional community phase over time. While oak savanna communities are dominated by grasses, these species can suppress forb establishment and reduce overall diversity and ecological functioning (Martin and Wilsey 2006; Williams et al. 2007). Reducing accumulated plant litter from the tall grasses allows more nutrients and light to become available for forb recruitment, allowing for greater ecosystem complexity (Wilsey 2008). Prescribed fire should be used on a cycle no less than every five years to allow the oaks to establish and mature (Gucker 2011).
Pathway 5.1A
Community 5.1 to 5.2
Selective herbicides are used to control non-native species, and prescribed fire and/or light grazing help to increase the native species diversity and control non-oak woody vegetation.
Pathway 5.2A
Community 5.2 to 5.1
Reconstruction experiences a decrease in native species diversity from drought or improper timing of management actions (e.g., reduced fire frequency, use of non-selective herbicides).
Transition T1A
State 1 to 2
Long-term fire suppression transitions the site to the fire-suppressed state (2).
Transition T1B
State 1 to 3
Vegetation removal and human alterations/transportation of soils transitions the site to the anthropogenic state (3).
Transition T1C
State 1 to 4
Tillage, seeding of agricultural crops, and non-selective herbicide transition the site to the cropland state (4).
Transition T2A
State 2 to 3
Vegetation removal and human alterations/transportation of soils transitions the site to the anthropogenic state (3).
Transition T2B
State 2 to 4
Tillage, seeding of agricultural crops, and non-selective herbicide transition this site to the cropland state (4).
Restoration pathway R2A
State 2 to 5
Site preparation, invasive species control, and seeding native species transition this site to the reconstructed savanna state (5).
Transition T4A
State 4 to 2
Land abandonment transitions the site to the fire-suppressed state (2).
Transition T4B
State 4 to 3
Vegetation removal and human alterations/transportation of soils transitions the site to the anthropogenic state (3).
Restoration pathway R4A
State 4 to 5
Site preparation, invasive species control, and seeding native species transition this site to the reconstructed savanna state (5).
Transition T5A
State 5 to 2
Fire suppression and removal of active management transitions this site to the fire-suppressed state (2).
Transition T5B
State 5 to 3
Vegetation removal and human alterations/transportation of soils transitions the site to the anthropogenic state (3).
Transition T5C
State 5 to 4
Tillage, seeding of agricultural crops, and non-selective herbicide transition this site to the cropland state (4).
Additional community tables
Interpretations
Supporting information
Inventory data references
No field plots were available for this site. A review of the scientific literature and professional experience were used to approximate the plant communities for this provisional ecological site. Information for the state-and-transition model was obtained from the same sources. All community phases are considered provisional based on these plots and the sources identified in this ecological site description.
Other references
Angel, J. No date. Climate of Illinois Narrative. Illinois State Water Survey, Prairie Research Institute, University of Illinois at Urbana-Champaign. Available at https://www.isws.illinois.edu/statecli/General/Illinois-climate-narrative.htm. Accessed 8 November 2018.
Asbjornsen, H., L.A. Brudvig, C.M. Mabry, C.W. Evans, and H.M. Karnitz. 2005. Defining reference information for restoring ecologically rare tallgrass oak savannas in the midwestern United States. Journal of Forestry 103: 345-350.
Barrett, S.W. 1980. Indians and fire. Western Wildlands Spring: 17-20.
Brudvig, L.A., C.M. Mabry, J.R. Miller, and T.A. Walker. 2007. Evaluation of central North American prairie management based on species diversity, life form, and individual species metrics. Conservation Biology 21: 864-874.
Cleland, D.T., J.A. Freeouf, J.E. Keys, G.J. Nowacki, C. Carpenter, and W.H. McNab. 2007. Ecological Subregions: Sections and Subsections of the Coterminous United States. USDA Forest Service, General Technical Report WO-76. Washington, DC. 92 pps.
Colandonato, M. 1992. Carya cordiformis. In: Fire Effects Information System [Online]. U.S. Department of Agriculture, Forest Service, Rocky Mountain Research Station, Fire Sciences Laboratory. Available at https://www.crs-feis.org/feis/. (Accessed 23 March 2018).
Fierer, N., J. Ladau, J.C. Clemente, J.W. Leff, S.M. Owens, K.S. Pollard, R. Knight, J.A. Gilbert, and R.L. McCulley. 2013. Reconstructing the microbial diversity and function of pre-agricultural tallgrass prairie soils in the United States. Science 342: 621-624.
Gucker, C.L. 2011. Quercus macrocarpa. In: Fire Effects Information System [Online]. U.S. Department of Agriculture, Forest Service, Rocky Mountain Research Station, Fire Sciences Laboratory. Available at https://www.crs-feis.org/feis/. (Accessed 23 March 2018).
Karol, P. and D.A. Wardle. 2010. How understanding aboveground-belowground linkages can assist restoration ecology. Trends in Ecology and Evolution 25: 670-679.
LANDFIRE. 2009. Biophysical Setting 4213940 North-Central Interior Oak Savanna. In: LANDFIRE National Vegetation Dynamics Models. USDA Forest Service and US Department of Interior. Washington, DC.
Leake, J., D. Johnson, D. Donnelly, G. Muckle, L. Boddy, and D. Read. 2004. Networks of power and influence: the role of mycorrhizal mycelium in controlling plant communities and agroecosystem functioning. Canadian Journal of Botany 82: 1016-1045.
Martin, L.M. and B.J. Wilsey. 2006. Assessing grassland restoration success: relative roles of seed additions and native ungulate activities. Journal of Applied Ecology 43: 1098-1110.
Martin, L.M. and B.J. Wilsey. 2012. Assembly history alters alpha and beta diversity, exotic-native proportions and functioning of restored prairie plant communities. Journal of Applied Ecology 49: 1436-1445.
NatureServe. 2018. NatureServe Explorer: An online encyclopedia of life [web application]. Version 7.1 NatureServe, Arlington, VA. Available at http://explorer.natureserve.org. (Accessed 13 January 2020).
Nowacki, G.J. and M.D. Abrams. 2008. The demise of fire and “mesophication” of forests in the eastern United States. BioScience 58: 123-138.
Peel, M.C., B.L. Finlayson, and T.A. McMahon. 2007. Updated world map of the Köppen-Geiger climate classification. Hydrology and Earth System Sciences 11: 1633-1644.
Pyne, S.J., P.L. Andrews, and R.D. Laven. 1996. Introduction to Wildland Fire, Second Edition. John Wiley and Sons, Inc. New York, New York. 808 pps.
Schwegman, J.E., G.B. Fell, M. Hutchinson, G. Paulson, W.M. Shepherd, and J. White. 1973. Comprehensive Plan for the Illinois Nature Preserves System, Part 2 The Natural Divisions of Illinois. Illinois Nature Preserves Commission, Rockford, IL. 32 pps.
Skinner, R.H. 2008. High biomass removal limits carbon sequestration potential of mature temperate pastures. Journal for Environmental Quality 37: 1319-1326.
Smith, D.D., D. Williams, G. Houseal, and K. Henderson. 2010. The Tallgrass Prairie Center Guide to Prairie Restoration in the Upper Midwest. University of Iowa Press, Iowa City, IA. 338 pps.
Society for Ecological Restoration [SER] Science & Policy Working Group. 2002. The SER Primer on Ecological Restoration. Available at: http://www.ser.org/. (Accessed 28 February 2017).
Taft, J.B., G.S. Wilhelm, D.M. Ladd, and L.A. Masters. 1997. Floristic Quality Assessment for vegetation in Illinois, a method for assessing vegetation integrity. Erigenia 15: 3-95.
Taft, J.B., R.C. Anderson, L.R. Iverson, and W.C. Handel. 2009. Chapter 4: Vegetation ecology and change in terrestrial ecosystems. In: C.A. Taylor, J.B. Taft, and C.E. Warwick (eds.). Canaries in the Catbird Seat: The Past, Present, and Future of Biological Resources in a Changing Environment. Illinois Natural Heritage Survey Special Publication 30, Prairie Research Institute, University of Illinois at Urbana-Champaign. 306 pps.
Teague, W.R., S.L. Dowhower, S.A. Baker, N. Haile, P.B. DeLaune, and D.M. Conover. 2011. Grazing management impacts on vegetation, soil biota and soil chemical, physical and hydrological properties in tall grass prairie. Agriculture, Ecosystems and Environment 141: 310-322.
Tirmenstein, D.A. 1991. Quercus alba. In: Fire Effects Information System [Online]. U.S. Department of Agriculture, Forest Service, Rocky Mountain Research Station, Fire Sciences Laboratory. Available at https://www.crs-feis.org/feis/. (Accessed 23 March 2018).
Tomer, M.D., D.W. Meek, and L.A. Kramer. 2005. Agricultural practices influence flow regimes of headwater streams in western Iowa. Journal of Environmental Quality 34:1547-1558.
Undersander, D., B. Albert, D. Cosgrove, D. Johnson, and P. Peterson. 2002. Pastures for Profit: A Guide to Rotational Grazing (A3529). University of Wisconsin-Extension and University of Minnesota Extension Service. 43 pps.
United States Department of Agriculture – Natural Resource Conservation Service (USDA-NRCS). 2006. Land Resource Regions and Major Land Resource Areas of the United States, the Caribbean, and the Pacific Basin. U.S. Department of Agriculture Handbook 296. 682 pps.
U.S. Environmental Protection Agency [EPA]. 2013. Level III and Level IV Ecoregions of the Continental United States. Corvallis, OR, U.S. EPA, National Health and Environmental Effects Research Laboratory, map scale 1:3,000,000. Available at http://www.epa.gov/eco-research/level-iii-andiv-ecoregions-continental-united-states. (Accessed 1 March 2017).
White, J. and M.H. Madany. 1978. Classification of natural communities in Illinois. In: J. White. Illinois Natural Areas Inventory Technical Report. Illinois Natural Areas Inventory, Department of Landscape Architecture, University of Illinois at Urbana/Champaign. 426 pps.
White, J. 1994. How the terms savanna, barrens, and oak openings were used in early Illinois. In: J. Fralisch, ed. Proceedings of the North American Conference on Barrens and Savannas. Illinois State University, Normal, IL.
Williams, D.A., L.L. Jackson, and D.D Smith. 2007. Effects of frequent mowing on survival and persistence of forbs seeded into a species-poor grassland. Restoration Ecology 15: 24-33.
Wilsey, B.J. 2008. Productivity and subordinate species response to dominant grass species and seed source during restoration. Restoration Ecology 18: 628-637.
Wisconsin Department of Natural Resources [WDNR]. 2015. The Ecological Landscapes of Wisconsin: An Assessment of Ecological Resources and a Guide to Planning Sustainable Management. Wisconsin Department of Natural Resources, PUB-SS-1131 2015, Madison, WI. 293 pps.
Contributors
Lisa Kluesner
Kristine Ryan
Sarah Smith
Tiffany Justus
Approval
Chris Tecklenburg, 4/22/2020
Acknowledgments
This project could not have been completed without the dedication and commitment from a variety of staff members. Team members supported the project by serving on the technical team, assisting with the development of state and community phases of the state-and-transition model, providing peer review and technical editing, and conducting quality control and quality assurance reviews.
List of primary contributors and reviewers.
Organization Name Title Location
Natural Resources Conservation Service Ron Collman State Soil Scientist Champaign, IL
Tonie Endres Senior Regional Soil Scientist Indianapolis, IN
Tiffany Justus Soil Scientist Aurora, IL
Lisa Kluesner Ecological Site Specialist Waverly, IA
Rick Neilson State Soil Scientist Indianapolis, IN
Jason Nemecek State Soil Scientist Madison, WI
Kevin Norwood Soil Survey Regional Director Indianapolis, IN
Kristine Ryan MLRA Soil Survey Leader Aurora, IL
Stanley Sipp Resource Inventory Specialist Champaign, IL
Sarah Smith Soil Scientist Aurora, IL
Chris Tecklenberg Acting Regional Ecological Site Specialist Hutchinson, KS
Rangeland health reference sheet
Interpreting Indicators of Rangeland Health is a qualitative assessment protocol used to determine ecosystem condition based on benchmark characteristics described in the Reference Sheet. A suite of 17 (or more) indicators are typically considered in an assessment. The ecological site(s) representative of an assessment location must be known prior to applying the protocol and must be verified based on soils and climate. Current plant community cannot be used to identify the ecological site.
Author(s)/participant(s) | |
---|---|
Contact for lead author | |
Date | 09/11/2022 |
Approved by | Chris Tecklenburg |
Approval date | |
Composition (Indicators 10 and 12) based on | Annual Production |
Indicators
-
Number and extent of rills:
-
Presence of water flow patterns:
-
Number and height of erosional pedestals or terracettes:
-
Bare ground from Ecological Site Description or other studies (rock, litter, lichen, moss, plant canopy are not bare ground):
-
Number of gullies and erosion associated with gullies:
-
Extent of wind scoured, blowouts and/or depositional areas:
-
Amount of litter movement (describe size and distance expected to travel):
-
Soil surface (top few mm) resistance to erosion (stability values are averages - most sites will show a range of values):
-
Soil surface structure and SOM content (include type of structure and A-horizon color and thickness):
-
Effect of community phase composition (relative proportion of different functional groups) and spatial distribution on infiltration and runoff:
-
Presence and thickness of compaction layer (usually none; describe soil profile features which may be mistaken for compaction on this site):
-
Functional/Structural Groups (list in order of descending dominance by above-ground annual-production or live foliar cover using symbols: >>, >, = to indicate much greater than, greater than, and equal to):
Dominant:
Sub-dominant:
Other:
Additional:
-
Amount of plant mortality and decadence (include which functional groups are expected to show mortality or decadence):
-
Average percent litter cover (%) and depth ( in):
-
Expected annual annual-production (this is TOTAL above-ground annual-production, not just forage annual-production):
-
Potential invasive (including noxious) species (native and non-native). List species which BOTH characterize degraded states and have the potential to become a dominant or co-dominant species on the ecological site if their future establishment and growth is not actively controlled by management interventions. Species that become dominant for only one to several years (e.g., short-term response to drought or wildfire) are not invasive plants. Note that unlike other indicators, we are describing what is NOT expected in the reference state for the ecological site:
-
Perennial plant reproductive capability:
Print Options
Sections
Font
Other
The Ecosystem Dynamics Interpretive Tool is an information system framework developed by the USDA-ARS Jornada Experimental Range, USDA Natural Resources Conservation Service, and New Mexico State University.
Click on box and path labels to scroll to the respective text.