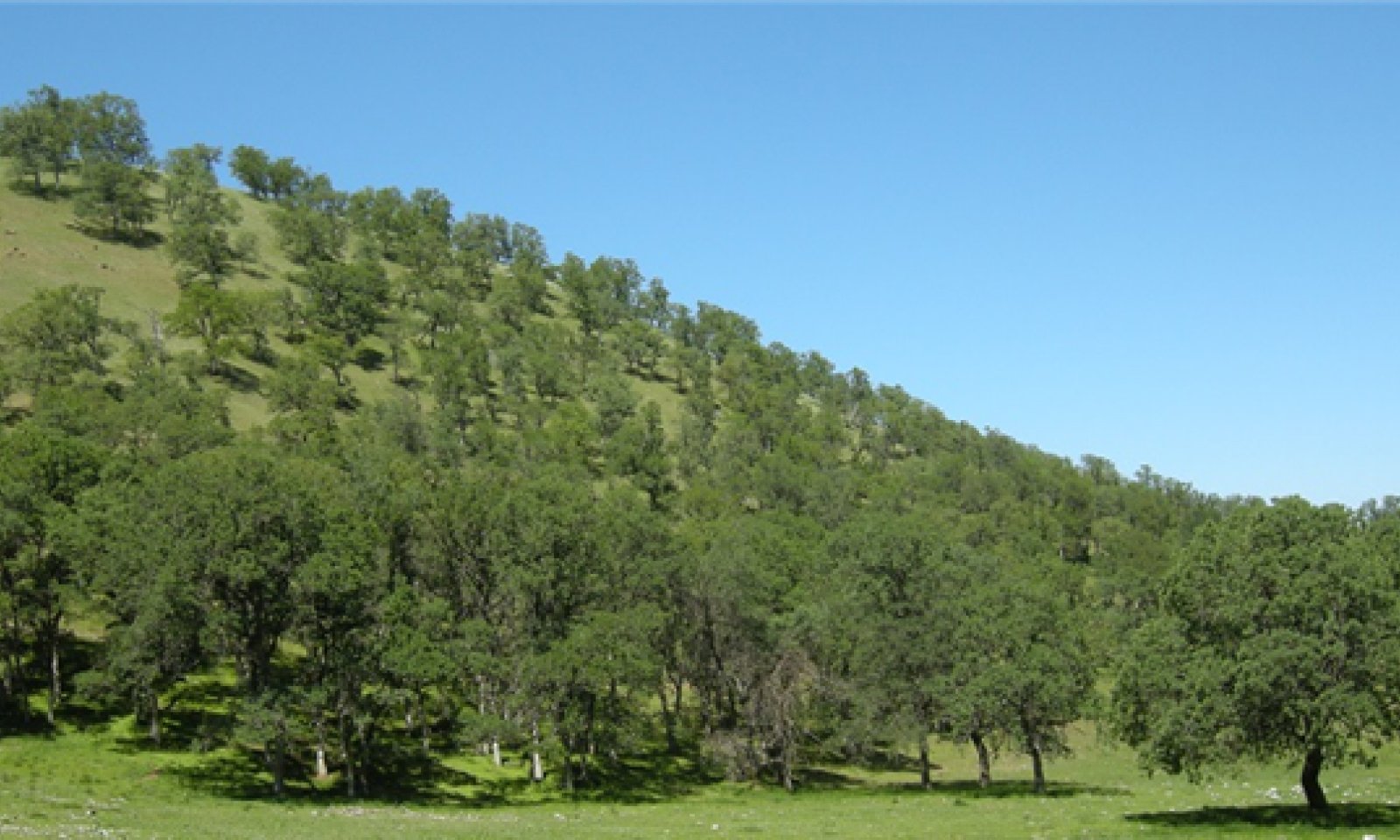

Natural Resources
Conservation Service
Ecological site R015XF002CA
Clayey Foothills
Accessed: 12/22/2024
General information
Approved. An approved ecological site description has undergone quality control and quality assurance review. It contains a working state and transition model, enough information to identify the ecological site, and full documentation for all ecosystem states contained in the state and transition model.
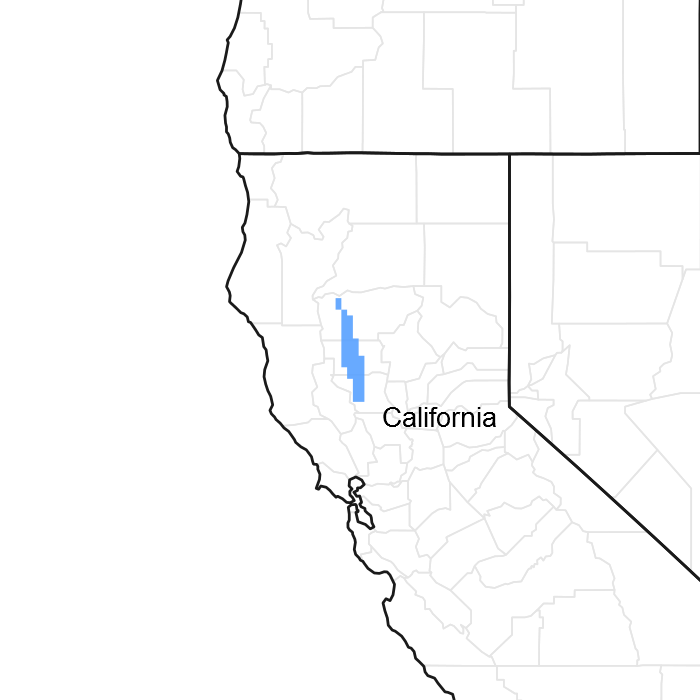
Figure 1. Mapped extent
Areas shown in blue indicate the maximum mapped extent of this ecological site. Other ecological sites likely occur within the highlighted areas. It is also possible for this ecological site to occur outside of highlighted areas if detailed soil survey has not been completed or recently updated.
MLRA notes
Major Land Resource Area (MLRA): 015X–Central California Coast Range
Major Land Resource Area (MLRA) 15: (Central California Coast Range)
Major Land Resource Area (MLRA) 15: This MLRA is an area of gently sloping to steep, low mountains. Precipitation is evenly distributed throughout fall, winter, and spring but is very low in summer. Elevation ranges from sea level to 2,650 feet (810 meters) in most of the area, but up to 4,950 feet (1,510 meters) in some of the mountains. The soils in the area dominantly have a thermic soil temperature regime, a xeric soil moisture regime, and mixed or smectitic mineralogy.
LRU Description:
This Land Resource Unit (designated by “15XF”) includes Blue Ridge in the northern California Coast Ranges and steep hills east of Blue Ridge and east of the Stony Creek fault, extending north to the Klamath Mountains down to the southern portion of Napa and Yolo Counties. The LRU is formed mostly from upper and lower Cretaceous sandstone, shale and conglomerate members of the Great Valley sequence. This area includes north to south trending foothill slopes and alluvial back valleys. Soil temperature regime is mostly thermic, with some high elevation areas that are mesic, and soil moisture regime is xeric. Common vegetation includes introduced annual grasses and forbs, blue oak, chamise, ceanothus, manzanita and California foothill pine. Elevations range from 1,000 to 2,400 feet. Rainfall levels drop quickly from the mountains to the foothills and valley due to the rain shadow effect. Annual precipitation generally averages from 16 to 40 inches. The Environmental Protection Agency (EPA) Ecoregion that this Ecological site is located in is designated as “6f”.
Classification relationships
This site may include the following Allen-Diaz Class: Blue Oak-Grass (Allen-Diaz et al., 1989). Blue Oak Woodland (BOW) of the California Wildlife Habitat Relationships System (Mayer and Laudenslayer, 1988). The Society for Range Management Cover Type (Shiflet (Ed.), 1994) for this site is Blue Oak Woodland. This site is includes the Quercus douglasii Woodland Alliance of the Manual of California Vegetation (Sawyer et al., 2009).
Ecological site concept
This ecological site is found on the eastern to southeast aspects of north to south trending steep backslopes and footslopes. The moderately deep to deep clayey soils associated with this ecological site have soil cracks that develop during late May and June and remain open until October or November when they are closed by winter rains. Precipitation ranges from 19 to 24 inches.
This ecological site is blue oak savanna with an understory dominated by non-native grasses, primarily oats (Avena spp.), both soft brome (Bromus hordeaceus) and red brome (Bromus rubens) and a variety of annual forbs and herbs. The overstory is blue oak (Quercus douglasii), with a low to moderate cover of whiteleaf manzanita (Arctostaphylos manzanita). Very scattered California foothill pine (Pinus sabiniana) may be found at higher elevations on this ecological site.
Associated sites
R015XF004CA |
Shallow Loamy Foothills Ecological site R015XF004CA, Shallow Loamy Foothills, is found on southeast to south trending foothill backslopes, shoulders and ridges with the LRU. Soils are shallow to soft or hard bedrock. Vegetation is annual grassland with a widely spaced blue oak overstory. |
---|
Similar sites
R015XF005CA |
Steep Loamy Foothills Ecological site R015XF005CA, Steep Loamy Foothills, predominantly occurs on moderately deep soils found on steep to very steep foothill shoulders and backslopes. Vegetation is moderate to heavy to shrubs with a blue oak and California foothill pine overstory. |
---|---|
R015XI009CA |
Deep Clay (Altamont) Ecological site R015XI009CA, Deep Clay (Altamont), is similar to this site but extends from Tehama to Santa Barbara County. |
R015XI001CA |
Clayey Hills Ecological site R015XI001CA, Clayey Hills, is similar to this site, but is found from Colusa to Yolo Counties at lower elevations. |
Table 1. Dominant plant species
Tree |
(1) Quercus douglasii |
---|---|
Shrub |
(1) Arctostaphylos manzanita |
Herbaceous |
(1) Avena fatua |
Physiographic features
This ecological site is found primarily on east and southeast flanks of north to south trending foothill backslopes and footslopes (see the physiographic diagram below). This site forms a "mosaic" pattern with an associated ecological site, R015XF004CA, Shallow Loamy Foothills. The ecological site is generally found on slopes greater than 30 percent, but overall slopes may range from 10-60 percent. These steep slopes may generate a very high degree of runoff during storm events. Typical elevations average from 700 to 1,300 feet but more broadly range from 300 to 1,500 feet. The lower elevational range of this site occurs in the adjacent EPA Ecoregion 6e.

Figure 2. Clayey Foothills - R015XF002CA
Table 2. Representative physiographic features
Landforms |
(1)
Hill
|
---|---|
Flooding frequency | None |
Ponding frequency | None |
Elevation | 213 – 396 m |
Slope | 30 – 60% |
Aspect | E, SE |
Climatic features
This ecological site has a Mediterranean climate characterized by hot summer temperatures and cool moist winters. The driest time of the year is June, July and August. Precipitation falls primarily as rain during October through May. The northern part of the California Central Valley receives precipitation from winter storms from the Pacific Northwest. The timing length and intensity of storms are highly variable and unpredictable.
Periodic drought may occur for months or years at a time, depending on the fluctuations of winds and ocean currents in the equatorial region of the Pacific Ocean (Quinn and Keeley, 2006). ]
The mean annual precipitation is 19 to 24 inches and the mean annual air temperature is 58 to 63 degrees Fahrenheit. The frost-free period averages 224 days. The freeze free period averages 279 days.
Two climate stations were used for this ecological site; East Park Reservoir and Stony George Reservoir. The northern extent of this ecological site tends to get higher precipitation.
Table 3. Representative climatic features
Frost-free period (average) | 224 days |
---|---|
Freeze-free period (average) | 279 days |
Precipitation total (average) | 610 mm |
Figure 3. Monthly precipitation range
Figure 4. Monthly average minimum and maximum temperature
Figure 5. Annual precipitation pattern
Figure 6. Annual average temperature pattern
Climate stations used
-
(1) EAST PARK RSVR [USC00042640], Stonyford, CA
-
(2) STONY GORGE RSVR [USC00048587], Elk Creek, CA
Influencing water features
A clayey soil texture, soil cracking, micro-topography and steep slopes affect water infiltration and runoff on this ecological site. Even though the soils are weakly vertic, water infiltration is still rapid into soil cracks until wetting then permeability is slow and runoff is low to very high. Soil creep noted on steep slopes (>30 percent) may indicate periods of saturated soils. Slope positions (concave downslope and convex across slope), steep slopes and heavy clay soil texture may contribute to a decrease in slope stability. On lower footslope positions this site experiences “run on” with higher clay accumulations and increased soil depth.
Soil features
The soils typically associated with this ecological site occur on steep footslopes and backslopes formed in residuum from Cretaceous sandstone and shale facies of the Great Valley sequence. Soils are moderately shallow to deep to a soft or hard bedrock contact.
Dominant soils are clayey throughout the soil profile. Surface textures are clay and clay loam and subsurface textures are clay. Clay content ranges from 35 to 60 percent. Water availability is moderate to high (4 to 7 inches) on these sites. There are no surface gravels. Subsurface gravels less than 3 inches by volume are predominantly 0-2 percent but range from 0 to 80 percent. Subsurface gravels greater than 3 inches by volume are predominantly are from 0 to 7 percent.
Soil cracking allows initial rapid water infiltration that decreases rapidly after soil wetting; permeability is thereafter slow and runoff is medium to very high. This soil characteristic is particularly common in the footslope positions of this ecological site. When these soils are wet they are susceptible to deformation under stress. The soil also has a high resilience when dry. Soil compaction from moderate to heavy grazing or grazing during wet periods may result in reduced water infiltration, and increased surface run-off causing soil erosion (Daniel et al., 2002). Slow permeability and steep slopes make this site susceptible to erosion if disturbed. Soil creep has been noted on steep slopes based on the observation of tree bole sweep.
Sehorn (Fine, smectitic, thermic Aridic Haploxererts), Altamont (Fine, smectitic, thermic Aridic Haploxererts).
This ecological site is correlated with the following map units and components in MLRA 15:
CA011; Colusa County, California:
218; Sehorn-Altamont Complex, 30 to 50 percent slopes; Sehorn and Altamont
241; Contra costa-Altamont association, 30 to 50 percent slopes; Altamont
329; Sehorn-Millsholm-Altamont complex, 15 to 30 percent slopes; Sehorn and Altamont
CA021; Glenn County, California:
AdE; Altamont Soils 30 to 65 percent slopes
AfsD; Altamont-Gullied land complex, shallow, 0 to 30 percent slopes
AfsE: Altamont-Gullied land complex, shallow, 30 to 65 percent slopes
SbC; Sehorn soils, 3 to 15 percent slopes
SdE; Sehorn-Millsholm, 30 to 65 percent slopes; Sehorn
CA645; Tehama County, California:
SmD; Sehorn-Millsholm complex, 10 to 30 percent slopes; Sehorn
SmE; Sehorn-Millsholm Complex, 30 to 50 percent slopes; Sehorn

Figure 7. Altamont Soils
Table 4. Representative soil features
Parent material |
(1)
Residuum
–
sandstone and shale
|
---|---|
Surface texture |
(1) Clay (2) Silty clay loam (3) Clay loam |
Family particle size |
(1) Clayey |
Drainage class | Well drained |
Permeability class | Slow |
Soil depth | 38 – 152 cm |
Surface fragment cover <=3" | 0% |
Surface fragment cover >3" | 0% |
Available water capacity (0-101.6cm) |
10.16 – 19.81 cm |
Calcium carbonate equivalent (0-101.6cm) |
0 – 1% |
Electrical conductivity (0-101.6cm) |
0 – 2 mmhos/cm |
Sodium adsorption ratio (0-101.6cm) |
0 – 2 |
Soil reaction (1:1 water) (0-101.6cm) |
6 – 8 |
Subsurface fragment volume <=3" (Depth not specified) |
0 – 2% |
Subsurface fragment volume >3" (Depth not specified) |
0 – 7% |
Ecological dynamics
Disturbance dynamics
Disturbance is defined as “any relatively discrete event in time that disrupts ecosystem, community, or population structure and changes resource pools, substrate availability, or the physical environment” (Pickett and White 1985); it may be natural or anthropogenic.
The interaction of several disturbance agents including fire, clearing, intensive agriculture, grazing, invasive species and drought has influenced and shaped the oak savanna and woodland environment.
Historic Influences
In the mid-1800s prior to major European settlement fire frequency was approximately every 25 years (McClaran 1986). Other authors indicate that the fire return interval was 5 to 15 years (Sawyer et al., 2009). Native Americans regularly used fire to manage vegetation communities to provide food and fiber (Blackburn and Anderson 1993; McCleary 2004). The historic vegetation community likely experienced an understory fire regime (Arno and Allison-Burnell, 2002). Frequent low intensity fire likely left widely spaced overstory trees and removed smaller trees and brush (McCleary, 2004). Following settlement before and after the gold rush (Pavlik 1991; Mensing 1992; Stephens 1997), fires were more frequent due to the intentional use of fire by ranchers and others to reduce brush. Shrub and oak recruitment increased in the absence of periodic fire or grazing in some environments (Purcell and Stephens, 2005). Active fire suppression during the last century has allowed for the accumulation of fuels and a trend towards larger more devastating fires (McCleary 2004; Arno and Allison-Bunnell, 2002).
Clearing of oaks occurred in many areas throughout the 1880’s for agriculture and livestock purposes (McCleary, 2004). Increased settlement resulted in the loss of oaks in the support of fuels for railroads, mines and steamships. After the Second World War, there was extensive conversion of woodland to pasture, and the inventory of hardwood forest types in California has decreased with widespread conversion from residential and commercial development. Within the State of California about 1.9 million acres of hardwoods and chaparral were reported to have been cleared in rangeland improvement projects (Bolsinger, 1988). Chaining of oaks occurred during the 1960’s in an attempt to provide for more grassland production. The production increase was short-lived (less than 10-20 years) and oak representation on some landscapes was altogether eliminated. Clearing may result in the permanent removal of oak from this site.
The lack of natural regeneration in some oak woodland has been attributed to many factors including herbivory, acorn predation, competition from annual grasses, and altered fire regimes (Fryer 2007; UC 2007; Sweitzer and Van Vuren 2002). Within this ecological site there is evidence, especially on the deep soils, that oaks are able to successfully regenerate and several age classes are represented.
During the late 1800’s a combination of intensive agriculture and an influx of exotic species are thought to have influenced a species conversion from native perennials grass to that of annual grasses and forbs in a relative short time period (Burcham 1957; Bartolome 1987; Baker 1989; Stromberg et al., 2007). Non-native grasses now have become naturalized in much of California. Introduced annual forbs and grasses have unique adaptations that give them a competitive advantage over native species. Some of these plant adaptations include high seed production, fast early season growth and the ability to set seed in drought years (Stromberg et al., 2007). Intensive year-round grazing by cattle impacted many soils during the late 1800’s, resulting in reduced vegetative cover and soil compaction in some areas.
Current Influences
Fire: Much of this ecological site has not burned in several decades. Blue oak can withstand low-intensity ground fires but is very susceptible to moderate to severe fire (Pavlik et al., 1991). Blue oak is considered a weak sprouter following fire, and sprouting declines with age (Burns and Honkala 1990, McDonald 1990). Small oaks are more likely to sprout than larger oaks and sprouting is better on moist sites than drier sites (McCreary, 2004). Oak sprouting following fire or cutting has been noted on the deep soils found in this ecological site. Whiteleaf manzanita lacks burls and reproduces primarily by seed (Stuart and Sawyer, 2001) that requires fire to break the hard seed coat. Viable manzanita seed may remain "banked" in the soil from 10 to 40 years (Abrahamson, 2014).
Drought: California grasslands experience an annual summer water deficit (Barbour and Major, 1977) as a result of the Mediterranean-influenced climate. This water deficit in combination with periodic drought can lead to changes in grassland species composition and production as a result of prolonged low water availability (Stromberg et al., 2007). The most recent drought period, now in its fourth year is unprecedented in California’s climate record (Griffin and Anchukaitis, 2014). Increased temperature and evaporation will likely have a significant effect on species composition and productivity on this site, favoring more droughty species, lowering oak seedling survival (Grünzweig et al., 2008) and lessening overall range production. Oaks are efficient water users; they are adapted to very low moisture conditions by virtue of their small leaf size, the regulation of water loss through the leaf stomata and by tapping into water below fractured rock (Baldocchi et al., 2007). Oak leaves may brown and drop prematurely during a drought year and fully recover the following year, potentially affecting the acorn crop, however, prolonged severe drought can result in mortality in oaks, especially on south facing slopes (Harper et al., 1991).
Harvesting: In studies examining oaks following harvest (McCleary, 2008) one third of the trees sprouted, however, subsequent sprout survival was significantly affected by browsing. In general, smaller diameter oaks (less than 12 inches in diameter) sprout more readily than larger oaks (Standiford, 2011) and sprouting is more common in moist environments than in dry sites (McCleary et al., 2002; McCreary 2004).
Grazing: Currently species composition and productivity of the annual-dominated grassland and understory grasses and forbs vary greatly within and between years and is greatly influenced by the timing and amount of precipitation and the amount of residual dry matter (George et al., 2001a). Grazing (or the lack of grazing) and drought influence the amount of residual dry matter, impacting peak standing crop the following year. Besides weather and temperature, oak canopy may affect forage yield; in the drier areas of California with low oak cover, oak canopy enhances forage, and in higher precipitation areas with greater oak cover, production is reduced (Connor, 1996). Tree shading may help reduce thermal stress in cattle; as temperature increases so do water requirements (Hahn, 1985, George et al., 2015.). Soil disturbance from grazing and burrowing animals and feral pigs continue to create new opportunities for exotic species invasion.
Disease and Insects: Blue oak is subject to the influence of disease and insects. Some diseases of blue oak damage the heartwood of the trunk and large limbs (McDonald, 1990; Hickman et al., 2011). A white pocket called Inonotus dryophilus causes rot in the heartwood of living oaks. The sulphur conk (Laetiporus sulphureus) causes a brown cubical rot also of the heartwood of living oaks. The hedgehog fungus (Hydnum erinaceum) and the artist’s fungus (Ganoderma applanatum) are also capable of destroying the heartwood of living oaks. A disease of blue oak roots, the shoestring fungus rot (Armillaria mellea) gradually weakens trees at the base until they fall. A white root rot caused by Inonotus dryadeus also has been reported on blue oak. The fruit tree leaf roller (Archips argyrospila) can cause significant defoliation and when combined with a multi-year drought may increase oak tree mortality rates (USDA, 2006).
Climate: In California‘s Mediterranean climate evaporative demand and rainfall are out of sync with one another (Miller et al., 2012). During peak demand in the spring, water is quickly depleted from the soil profile and grasses senesce. After that period the only moisture available to woody plants is through root access to groundwater. Groundwater has been shown to be a critical link to blue oak survival over the prolonged summer drought period (Miller et al., 2010). Extended periods of drought could slow recovery and affect carbon uptake, hindering reproductive processes, leading to a reduction in seedling establishment.
The future influence of climate change on vegetation has been widely debated. Some climate models indicate that decreasing precipitation and increasing temperature could result in a potential shift in the blue oak type to the north and shrinking of the overall range of the species. This change in range is thought to be a potential result of increasing moisture stress with changing climate (Kueppers et al., 2005). Although there are many other factors that influence plant communities, climate related effects include the potential for a changed fire regime and more favorable conditions for species invasions (Stromberg et al., 2004).
State and transition model

Figure 8. Clayey Foothills - R015XF002CA
More interactive model formats are also available.
View Interactive Models
More interactive model formats are also available.
View Interactive Models
Click on state and transition labels to scroll to the respective text
State 1 submodel, plant communities
State 2 submodel, plant communities
State 3 submodel, plant communities
State 1
Reference State
The Reference State represents the natural range of variability for this ecological site. Periodic drought, grazing, clearing and fire are the most dominant disturbances influencing this site. States and Community Phases included in this document include those previously recognized by Fire and Resource Assessment Program (FRAP, State of California, 1998) and other entities, as a result of the use of ordination software and professional consensus (Allen-Diaz et al., 1989; Vayssieres and Plant, 1998; George et al., 1993). The reference state has two community phases: 1) Blue oak//Annual Grasses Phase: The lower elevations of this site tends to be grass and blue oak dominated. 2) Blue oak//Whiteleaf manzanita//Wild oat: With increasing elevation and slope tree and shrub density increases. Oaks help retain more water on site than grasslands (Baldocchi et al., 2007) and enhance soil quality through nutrient cycling, organic matter deposition and reduced soil bulk density (Dalgren et al., 2003). Blue oak contributes to soil productivity by increasing soil nutrition under trees through the continuous cycling of nutrients to the soil surface and their slow release by microbial decomposition (O’Geen et al., 2010). This recycling of nutrients provides an energy source to microbes, insects and other plants present on the site, increasing its productivity. As a result, soil and herbaceous vegetation under oak canopies have higher nitrogen and carbon resources than adjacent grasslands and appear to increase rates of soil nitrogen turnover, as well as increased rates of microbial activity (Herman et al., 2003). Trees increase the soil water holding capacity of savanna soils (Baldocchi et al., 2004). Soils under oaks retain water into the early summer months as ground temperatures are moderated by shading. Findings from this study also indicated that oak savannas retain and store more energy than grasslands, due to lower reflectance and surface temperatures. This state is relatively stable unless tree removal occurs. Research indicates that oak removal results in a rapid decline in soil quality, including a loss in soil organic matter and nitrogen through leaching (Dalgren et al., 2003; Herman et al., 2003; ucanr.edu, 2007). The duration of vegetation successional stages varies greatly, and is lacking detailed study, but it is estimated by some authors to take at least 50 years (Mayer and Laudenslayer, 1988). Early growth of oak is slow and then more rapid up to the age of 40 years. Most stands of blue oak range from 80 to 100 years of age (Kertis et al., 1993). Remnant older blue oak specimens found in more remote or steep locations may range up to 450 years of age (Stahle et al., 2013). Mature brush development can take 10 to 15 years. Blue oak and manzanita provide important breeding and foraging habitat during the winter and spring and their acorns and berries provide a vital food source for birds and mammals.
Community 1.1
Blue oak/Annual Grasses and Forbs
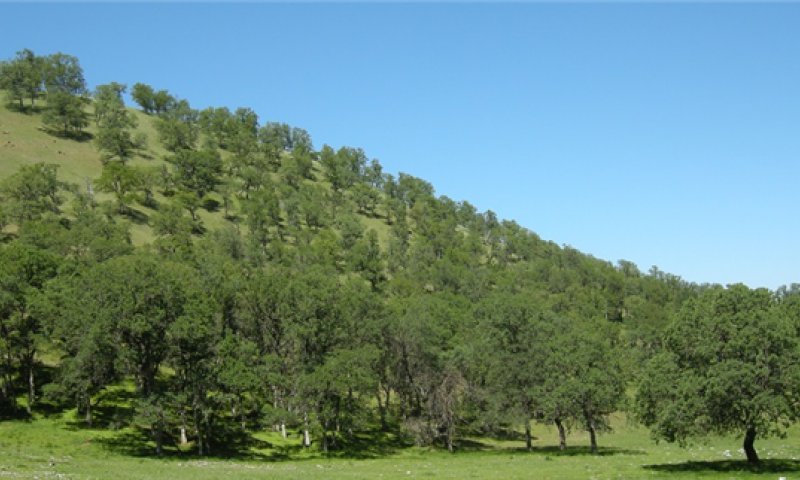
Figure 9. Footslope position - Community Phase 1.1 on Sehorn (Sde) soil. J. Welles 2014
Community Phase 1.1 of this ecological site is dominantly non-native grasses including oats (Avena fatua or Avena barbata) with a minor amount of desert fescue (Festuca microstachys). Common forbs and herbs may include springbeauty (Claytonia spp.), knotted hedgeparsley (Torilis nodosa), common chickweed (Stellaria media), longbeak stork’s bill (Erodium botrys), Ithuriel’s spear (Triteleia laxa) and miniature lupine (Lupinus bicolor). Blue oak (Quercus douglasii) dominates the overstory. This community phase is most commonly found on the lower footslope positions of this ecological site. These locations often have higher production than the other community phase in the Reference State due to increased nutrient accumulation and water run on from upper slopes.
Figure 10. Annual production by plant type (representative values) or group (midpoint values)
Table 5. Annual production by plant type
Plant type | Low (kg/hectare) |
Representative value (kg/hectare) |
High (kg/hectare) |
---|---|---|---|
Grass/Grasslike | 482 | 807 | 1300 |
Tree | 314 | 785 | 942 |
Forb | 45 | 504 | 717 |
Total | 841 | 2096 | 2959 |
Table 6. Ground cover
Tree foliar cover | 0-1% |
---|---|
Shrub/vine/liana foliar cover | 0-1% |
Grass/grasslike foliar cover | 0-1% |
Forb foliar cover | 0-1% |
Non-vascular plants | 0-3% |
Biological crusts | 0% |
Litter | 79-80% |
Surface fragments >0.25" and <=3" | 0% |
Surface fragments >3" | 0% |
Bedrock | 0% |
Water | 0% |
Bare ground | 15-20% |
Community 1.2
Blue oak//Whiteleaf manzanita//Wild oat
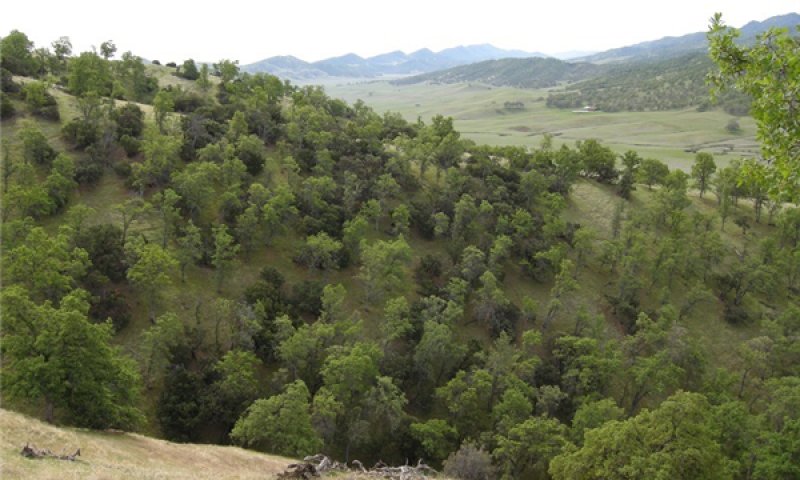
Figure 11. Community Phase 1.2 on Altamont soil. J.Welles 2013.
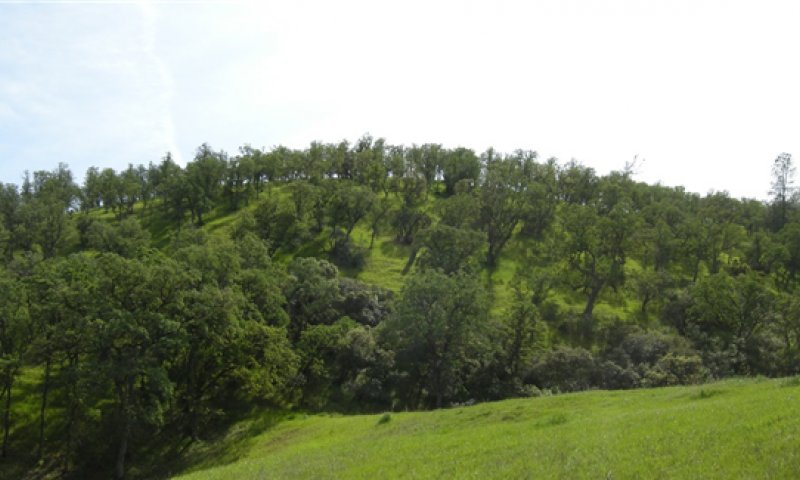
Figure 12. Reference Community Phase 1.2 Landscape. Welles, 2015
This community phase is both the representative and reference community representing the ecological potential for this site. The reference community phase is oak savanna with an understory dominated by non-native grasses, primarily wild oat or slender oat (Avena fatua and Avena barbata), and minor amounts of desert fescue (Vulpia microstachys) soft brome (Bromus hordeaceus), Spanish brome (Bromus madritensis) perennial ryegrass (Lolium perenne) and red brome (Bromus rubens). Annual forbs and herbs include knotted hedgeparsley (Torilis nodosa), little burclover (Medicago minima) and dovefoot geranium (Geranium molle). Perennial forbs and herbs include straightbeak buttercup (Ranunculus orthorhynchus), blue dicks (Dichelostemma capitatum), and onion (Allium spp.).The overstory is blue oak (Quercus douglasii), with a sparse to moderate shrub cover of whiteleaf manzanita (Arctostaphylos manzanita). Very scattered foothill pine (Pinus sabiniana) may be found at higher elevations on this ecological site. The shrub cover in the reference state helps to slow the water runoff rate. The improved water storage under shrubs and trees makes for plant available water later in the growing season due to decreased evaporation and shading, maintaining water longer as opposed to just grasses (Gill and Burke, 1999). Some deeply rooted trees and shrubs may also induce hydraulic lift, transporting water to the upper soil layers (Richards and Cadewell, 1987; Caldwell et al., 1998, Ishikawa and Bledsoe, 2000; Liste and White, 2008), supporting the development of neighboring plants. Nutrients are also concentrated around shrub bases from litter fall and from sediment capture via movement of soil particles. The increase in shrub cover causes a reduction in the amount of grass and forb cover as compared to community phase 1.1. Reference community phase production: Expected production is highly variable based on unfavorable normal or favorable year. Drought over the last several years has hampered efforts to sample the full range of site conditions. Total production in a 50-80 percent of normal years ranges from a low of 600 pounds per acre to a high of 1,800 pounds per acre. Historic Range Site data indicates a range of 950 to 2,400 lbs/acre for measured grass and forb production.
Figure 13. Annual production by plant type (representative values) or group (midpoint values)
Table 7. Annual production by plant type
Plant type | Low (kg/hectare) |
Representative value (kg/hectare) |
High (kg/hectare) |
---|---|---|---|
Grass/Grasslike | 493 | 824 | 1317 |
Tree | 151 | 381 | 527 |
Forb | 11 | 78 | 101 |
Shrub/Vine | 22 | 67 | 78 |
Total | 677 | 1350 | 2023 |
Table 8. Ground cover
Tree foliar cover | 0-1% |
---|---|
Shrub/vine/liana foliar cover | 1-2% |
Grass/grasslike foliar cover | 1% |
Forb foliar cover | 0-1% |
Non-vascular plants | 0-1% |
Biological crusts | 0-1% |
Litter | 38-51% |
Surface fragments >0.25" and <=3" | 0-1% |
Surface fragments >3" | 0-1% |
Bedrock | 0% |
Water | 0% |
Bare ground | 10-40% |
Figure 14. Plant community growth curve (percent production by month). CA1501, Annual rangeland (Normal Production Year). Growth curve for a normal (average) production year resulting from the production year starting in November and extending into early May. Growth curve is for oak-woodlands and associated annual grasslands..
Jan | Feb | Mar | Apr | May | Jun | Jul | Aug | Sep | Oct | Nov | Dec |
---|---|---|---|---|---|---|---|---|---|---|---|
J | F | M | A | M | J | J | A | S | O | N | D |
0 | 10 | 25 | 40 | 5 | 0 | 0 | 0 | 0 | 0 | 10 | 10 |
Figure 15. Plant community growth curve (percent production by month). CA1502, Annual rangeland (Favorable Production Year). Growth curve for a favorable production year resulting from the production year starting in October and extending through May. Growth curve is for oak-woodlands and associated annual grasslands..
Jan | Feb | Mar | Apr | May | Jun | Jul | Aug | Sep | Oct | Nov | Dec |
---|---|---|---|---|---|---|---|---|---|---|---|
J | F | M | A | M | J | J | A | S | O | N | D |
0 | 10 | 20 | 30 | 25 | 0 | 0 | 0 | 0 | 5 | 5 | 5 |
Figure 16. Plant community growth curve (percent production by month). CA1503, Annual rangeland (Unfavorable Production Year). Growth curve for an unfavorable production year resulting from the production year starting in October and extending through May. Growth curve is for oak-woodlands and associated annual grasslands..
Jan | Feb | Mar | Apr | May | Jun | Jul | Aug | Sep | Oct | Nov | Dec |
---|---|---|---|---|---|---|---|---|---|---|---|
J | F | M | A | M | J | J | A | S | O | N | D |
0 | 15 | 70 | 5 | 0 | 0 | 0 | 0 | 0 | 0 | 5 | 5 |
Pathway 1.1A
Community 1.1 to 1.2
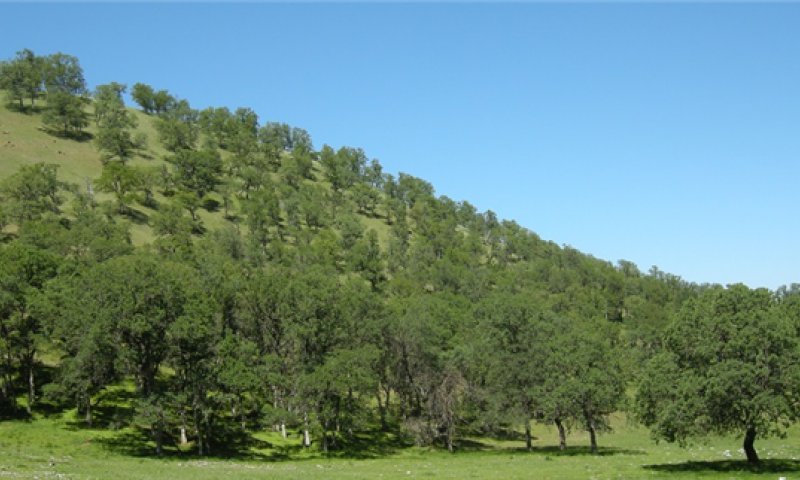
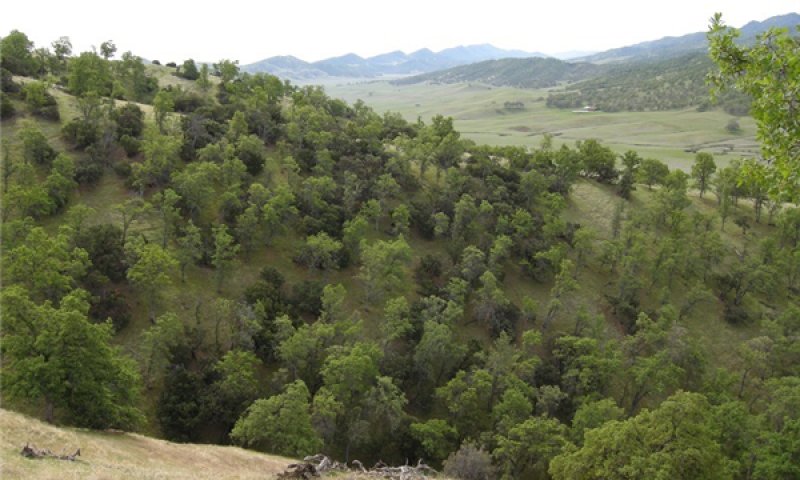
Whiteleaf manzanita (Arctostaphylos manzanita) presence on this ecological site increases on north and east-facing slopes, presumably due to reduced competition from grasses and favorable soil moisture conditions. This shrub lacks burls and reproduces primarily by seed (Stuart and Sawyer, 2001) following moderate fire and occasionally from equipment disturbance that scarifies the hard seed coat. Manzanita shrubs may store large amounts of "banked" seed in the soil that may remain viable for 10 to 40 years. Their seed can be spread by mammals that eat the manzanita fruit or berries (Abrahamson, 2014).
Pathway 1.2A
Community 1.2 to 1.1
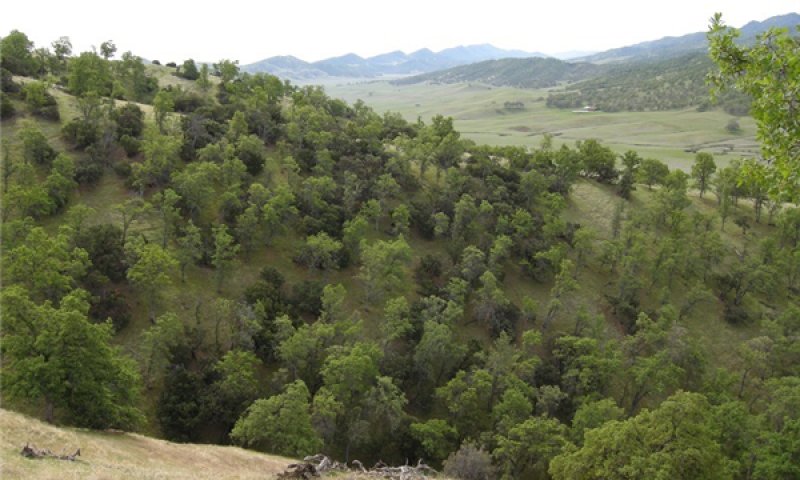
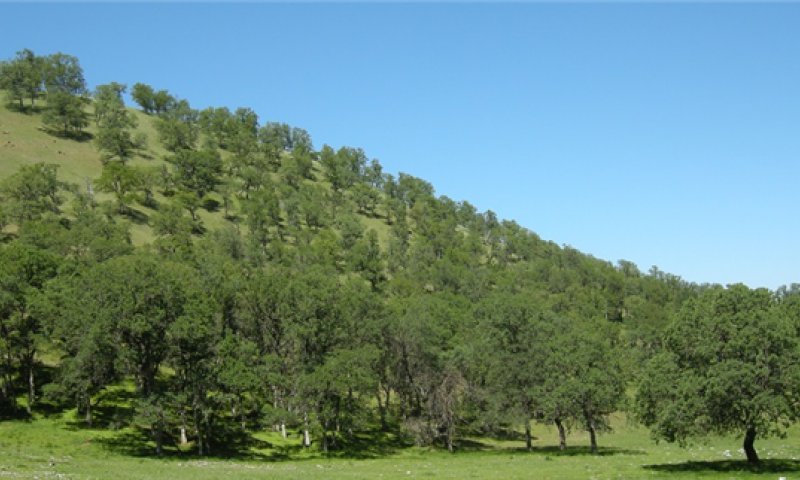
Natural regeneration of shrubs is largely fire-dependent and the lack of fire has resulted in shrub decadence and low recruitment. Whiteleaf manzanita is an obligate seeders, requiring fire for seed germination that is enhanced by scarification and charate (Abrahamson, 2014). Mechanical treatment could also eliminate manzanita and return this to a grass-dominated plant community, although mechanical removal and grazing may result in lower rates of water infiltration and higher runoff (Daryanto and Eldridge, 2010).
State 2
Annual Grassland State
Today species composition and productivity of the annual dominated understory grasses and forbs vary greatly within and between years and is greatly influenced by the timing and amount of precipitation and the amount of residual dry matter (George et al., 2001a; Conner, 1991). Residual mulch influences and impacts germination and organic matter (George et al., 1985). Lower footslope positions may support more grasses than trees in some areas of heavy clay accumulations, as increased soil cracking may destroy woody roots restricting shrub or tree growth (Stromberg et al., 2007). This cracking affects dynamic soil properties by allowing for initial rapid water infiltration that decreases rapidly after soil wetting; permeability is thereafter slow and runoff is medium to very high, increasing the potential for erosion. Nutrient turnover is rapid in grassland systems and is lost via leaching, gaseous exchange and soil erosion (Stromberg et al., 2007; ucan.edu, 2014). Because most of the nitrate that accumulates during the summer and fall is moved to seeds at senescence and the remainder is removed via rains prior to initiation of growth, little is available for later absorption by growing plants. Annual systems with shallow root systems dry out quickly with rapid spring growth and evapotranspiration quickly depletes soil moisture (ucan.edu, 2014).
Community 2.1
Annual Grass-Dominated
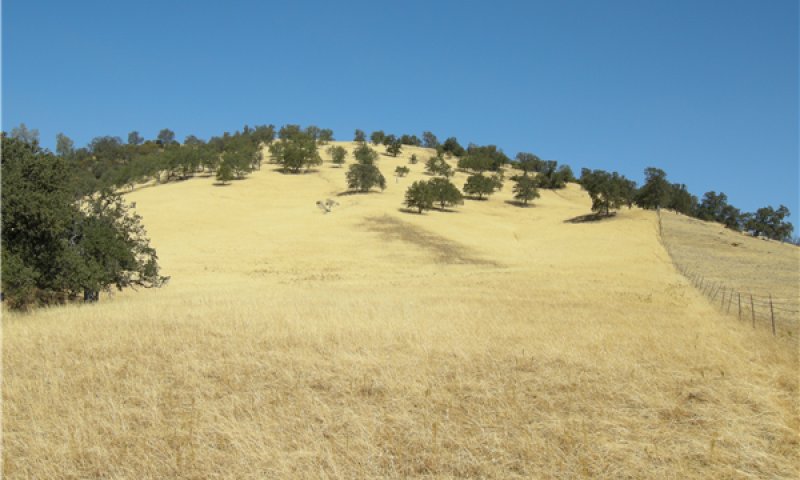
Figure 17. Community Phase 2.1 in foreground on Sehorn Soil. J. Welles, 2013.
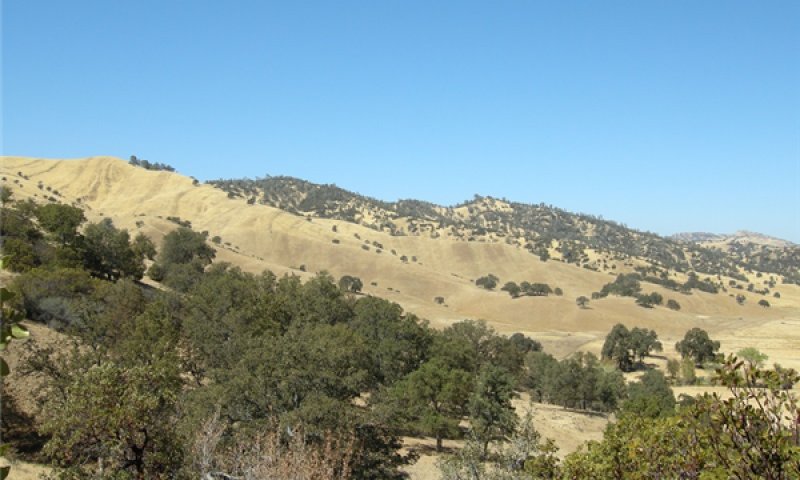
Figure 18. Community Phase 2.1. Cleared site in midground on Altamont soil. J. Welles, 2013
Community Phase 2.1 of this ecological site is dominantly non-native annual grasses including oats (Avena spp.) and bromes (Bromus spp.) Other common annual grasses, forbs and herbs include soft brome (Bromus hordeaceus), Spanish brome (Bromus madritensis), bluedicks (Dichelostemma capitatum), and pincushionplant (Navarretia spp.). Yellow star-thistle (Centauria solstitialis) and medusahead (Taeniatherum caput-medusae) are also present though generally sparsely represented on the higher slope positions. Sufficient litter or residue is required for good germination of grass species (Young et al., 1981) and leaving greater amounts may favor grass dominance (George et al., 1985). Residue or mulch improves soil fertility and increases infiltration as well (Heady, 1956). Minimum residual dry matter (RDM) guidelines for dry annual grassland suggest retention of 300 to 600 pounds per acre, with greater retention as slope increases (Bartolome et al., 2002) to provide for soil and nutrient retention. Litter improves soil fertility and increases infiltration as well by providing cover during the hot summers, reducing evapotranspiration rates, leaving more moisture in the soil profile (Heady, 1956). Grasses have positive effects on soils by enhancing water percolation, aeration and carbon storage (Eviner and Chapin, 2001). Certain grasses that produce high amounts of litter such as wild oat and soft brome may attract voles and mice.
Community 2.2
Annual Legume-Dominated
Notchleaf clover (Trifolium bifidum), rose clover (Trifolium hirtum) or sky lupine (Lupinus nanus) may be found on this site and other clovers and lupines may also be present. Leguminous species like clovers (Trifolium spp.), and lupines (Lupinus spp.) are important nitrogen-fixing plant species as they are capable of increasing forage yield where they are in a mixed cover crop with grasses. Legumes increase fertility but may also attract gophers (Eviner and Chapin, 2001). Nitrogen availability is highest in the fall when there is potential for losses through leaching.
Community 2.3
Forb-Dominated
Filaree spp., either longbeak stork’s bill (Erodium botrys) or redstem stork’s beak (Erodium cicutarium) may be present on this site. Filaree years occur in low rainfall years or when residual dry matter (Bartolome et al., 2002) is low. Drought, heavy grazing and fire are triggers may all result in filaree or forb dominated understory. Rates of nitrogen mineralization of filaree are low due to their concentration of phenolics that reduce the rate of breakdown of plant material (Eviner and Chapin, 2001). Following a fire filaree may dominate the site for up to three years (Parsons and Stohlgren, 1989; McDougald et al., 1991). Often when a dry period follows the first rains, drought tolerant seedlings are favored. Spring or early summer burns can increase the incidence of filaree as opposed to fall burns.
Pathway 2.1A
Community 2.1 to 2.2
Legume dominated years may be triggered when rains fall later in the season (Kreitlow and Hart 1974) and following fire (D’Antonio et al, 2006).
Pathway 2.1B
Community 2.1 to 2.2
Filaree years occur in low rainfall years or when residual dry matter (Bartolome et al. 2002) is low. Drought, heavy grazing and fire are triggers that may result in filaree or forb dominated understory. Following a fire filaree may dominate the site for up to three years (Parsons and Stohlgren 1989, McDougald et al, 1991). Often when a dry period follows the first rains, drought tolerant seedlings are favored. Spring or early summer burns can increase the incidence of filaree as opposed to fall burns.
Pathway 2.2B
Community 2.2 to 2.1
Grass dominated years are triggered when rainfall is well-distributed or greater than normal and when residual dry matter is high due to favorable weather or light grazing pressure (George, 2001b).
Pathway 2.2A
Community 2.2 to 2.3
Filaree years occur in low rainfall years or when residual dry matter (Bartolome et al., 2002) is low. Drought, heavy grazing and fire are triggers may result in filaree or forb dominated understory. Following a fire filaree may dominate the site for up to three years (Parsons and Stohlgren 1989, McDougald et al, 1991). Often when a dry period follows the first rains, drought tolerant seedlings are favored. Spring or early summer burns can increase the incidence of filaree as opposed to fall burns.
Pathway 2.3A
Community 2.3 to 2.1
Grass dominated years occur when rainfall is well-distributed or greater than normal. Range planting could be utilized to alter or enhance species composition.
Pathway 2.3B
Community 2.3 to 2.2
Legume dominated years may be favored when rains fall later in the season (Kreitlow and Hart, 1974) and following fire (D’Antonio, et al., 2006).
State 3
Noxious Weed-Dominated State
The two dominant invasive species that occur in this state (Cal-IPC List 1A) include medusahead (Taeniatherum caput-medusae) and yellow star-thistle (Centaurea solstitialis). These plants are commonly found in greater abundance in the lower footslope positions due to the clayey nature of the soils; though they are also found on backslopes but are not nearly as common. Italian plumeless thistle (Carduus pychnocephalus, Cal-IPC list 2C) may also be found in limited populations but is not as extensive as the other above-mentioned species. The California Invasive Plant Council (Cal IPC http://www.cal-ipc.org/) maintains an invasive plant inventory that rates invasive plants based on their ecological impacts to native flora and fauna. The California Department of Food and Agriculture (CDFA) also maintain a list of "noxious weeds" that are subject to regulation or quarantine by county agricultural departments. Some experts have suggested that medusahead and other invasive species may gradually adapt to new sites (Rice et al., 2006).
Community 3.1
Medusahead
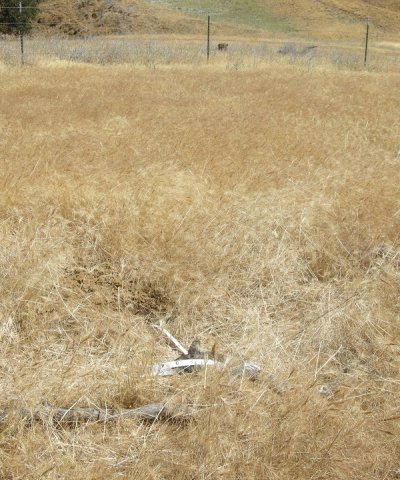
Figure 19. Medusahead on Altamont footslope. J. Welles, 2012
Medusahead competes successfully with other plants by going to seed in late spring, avoiding competition for remaining soil moisture (Kyser et al., 2014). A prolific seeder, most medusahead seed falls near the plant but is also spread via animals and human activity. The plant’s high silica content creates a dense litter that ties up nutrient cycling, slows soil warming in spring and prevents seed penetration of other grass species (DiTomaso et al., 2007). Medusahead seeds are adapted to germinate in and under its own litter (DiTomaso et al., 2006). Heavy thatch suppresses most other herbaceous species and poses a fire hazard in summer months. Medusahead can reduce grazing capacity by 75 to 80 percent (Kyser et al., 2014). Late maturity of medusahead in relation to other annual grasses and its subsequent requirements for high water-holding capacity clay soils determines its abundance. Medusahead acquire soil nutrients more easily than other grasses, accelerating its growth rate and making soils with high nutrient levels more susceptible to invasion (Kyser et al., 2014).
Community 3.2
Yellow star-thistle
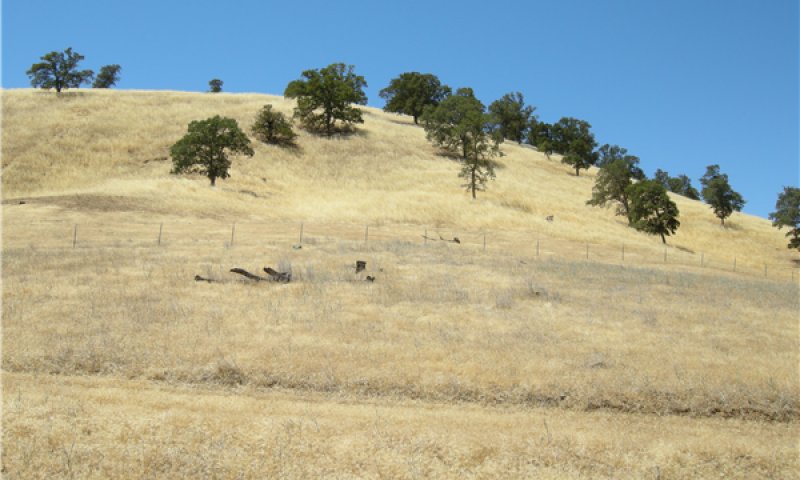
Figure 20. Yellow star-thistle footslope on Altamont soil. Welles, 2013
High germination rates and access to water from deep roots (>3 feet in depth) enable yellow star-thistle to out-compete most all rangeland plants (DiTomaso et al., 2006). On deep soils water availability extends late into the growing season, reducing competition at the reproductive stage. Yellow star-thistle has been shown to deplete soil moisture reserves and alter water cycles in annual grasslands (Holloran, 2004). Depletion of moisture in the soil profile is equivalent to a reduction of 15 to 25 percent of precipitation (Jetter et al., 2003). Infestations can reduce livestock carrying capacity by 10 to 50 percent (DiTomaso et al., 2006). This deep-rooted species has access to water later in the season and may affect nitrogen cycling and fluxes, with peak nitrogen cycling occurring later in the season and at different depths than annual grasses (Stromberg et al., 2004).
Pathway 3.1A
Community 3.1 to 3.2
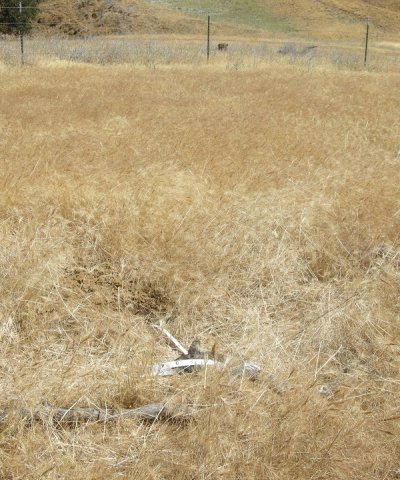
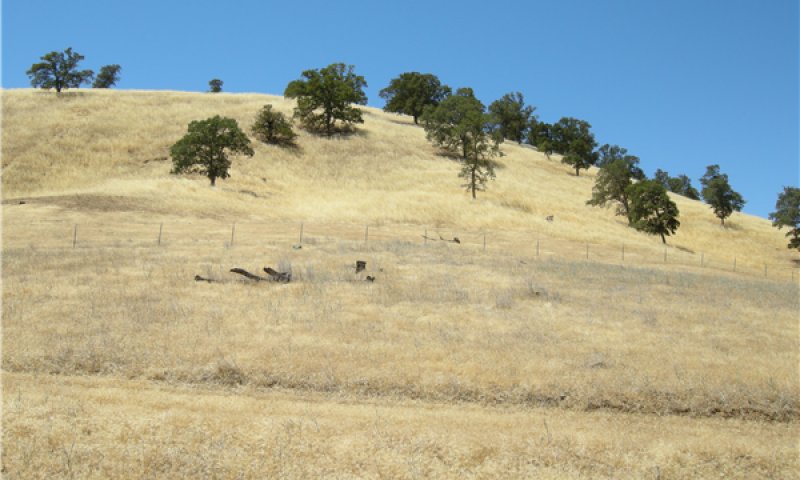
Yellow star-thistle invasion increases with disturbance and seed is often spread by vehicles or the transportation of livestock (DiTomaso et al., 2007) but it may also arrive as a contaminant in grass hay. Animals and humans are responsible for seed transported by adherence to fur and clothing (DiTomaso et al., 2006).
Pathway 3.2A
Community 3.2 to 3.1
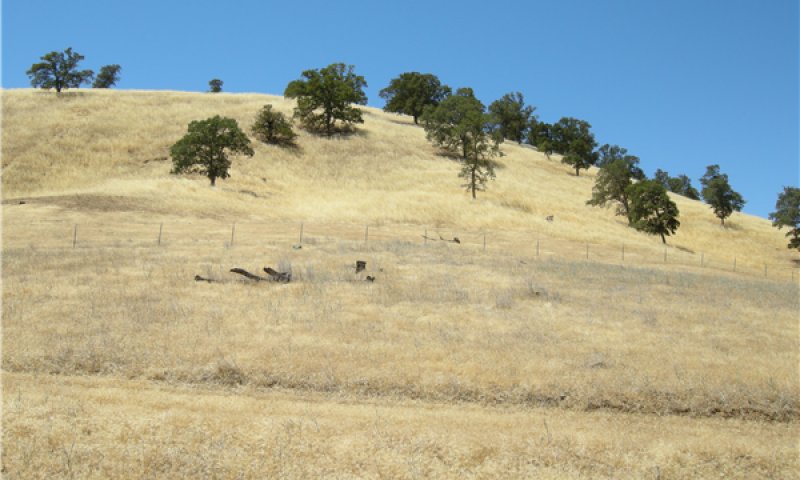
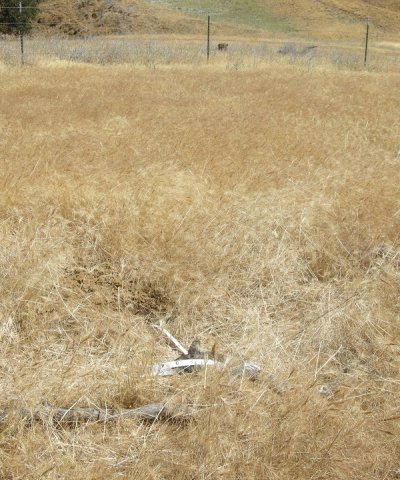
Medusahead seed had long awns with silica scales (Kyser et al, 2014) that easily attach to animals, clothing, vehicles and machinery; long distance dispersal has been connected to travel routes.
Transition T1A
State 1 to 2
Triggers that limit blue oak reproduction and or survival (intensive prolonged grazing) in combination with tree removal (severe fire, clearing or type conversion), or prolonged drought resulting in tree mortality could put this community phase at risk, eventually reaching a threshold (T1A) where it becomes an annual grassland state (State 2). Reduction of oak canopy below 20 percent could result in a reduction in oak regeneration and survival (FRAP, State of California, 1998); natural regeneration seldom occurs further away than 100 feet from an existing tree canopy and stump sprouting is not likely in mature trees in a low rainfall area. Blue oak seedlings may be less adapted to drought conditions, affecting their growth and survival (Grünzweig et al., 2008). As older trees die and there are no seedling replacements, the lack of seedlings could lead to conversion to savanna to pasture (Baldocchi, 2004). One study (McCreary et al., 2008) found sprout survival 19 years following harvest was 28 percent and noted that sprouting would be expected to decline with any subsequent harvest. Removal of blue oaks has been found to reduce soil productivity due to decreased soil nutrition from tree litter (Dalgren et al., 2003), and increase the potential for erosion due to a decline in soil porosity and increased bulk density from organic matter losses (Dalgren et al., 1997). Erosion losses also may reduce productivity by changing the water-holding capacity of the soil and the thickness of the root zone (Elliot et al., 1998). Removal of trees or other vegetation may change the hydrology of an area, increasing runoff and reducing infiltration. Slope stability and erosion were increased after removal of stabilizing vegetation on steep slopes. Burning also increased sedimentation rates following conversion from oaks to grassland (Dahlgren et al., 2001).
Restoration pathway R2A
State 2 to 1
Natural regeneration of blue oaks may be limited on some drier sites and because of a number of factors that limit seed germination, seedling establishment and survival to the tree stage. Competition for soil moisture from the understory annual plants, acorn and seedling damage by rodents, livestock grazing and changed fire regimes are important factors that can reduce blue oak regeneration. Oak restoration can be difficult and expensive but limited success may be achieved through planting acorns or seedlings and protecting existing young seedlings or saplings. Regeneration studies conducted at the University of California Sierra Foothill Research and Extension Center (SFREC) were successful on rocky clay loam soils, however, average rainfall is lower on this ecological site (19-24 inches) than in the study area (28 inches), and supplemental irrigation may be necessary (McCreary, 2001), limiting this treatment to areas accessible by road. Young oak seedlings are especially susceptible to mortality during the early years. One study indicates blue oak seedlings that reach 10 years of age are more likely to survive compared to newly germinated seedlings (Philips et al., 2007). Protection of saplings from grazing pressure utilizing tree shelters (McCreary and George, 2005) or exclosures (Philips et al., 2007) has been shown to be an effective management technique to aid survival and accelerate growth of young seedlings. On this ecological site, the lower slopes may have more favorable moisture conditions and options for accessibility, however on most of the deeper soils associated with this ecological site appear to acceptable levels of oak regeneration and survival taking place. McCreary (2001) provides an extensive review of oak regeneration problems and practices on California’s oak woodlands.
Transition T2A
State 2 to 3
Various triggers (disturbances) that reduce site resilience lead to an increased potential for medusahead invasion and expansion include a lack of grazing and an absence of fire. A nearly continuous canopy of medusahead and a build-up of litter (high in silica) will exclude most other herbaceous species. A lack of grazing and absence of fire insures the dominance of medusahead. Triggers for yellow star-thistle invasion include disturbances such as intense grazing, poorly timed mowing, and rooting by feral pigs (DiTomaso et al, 2007). In areas with reduced plant cover and an increase in bare ground, opportunities are provided for invasion of noxious weeds like yellow star-thistle. The lower footslope positions with a deeper soil profile and higher clay accumulations are especially at-risk for a transition to a noxious weed state. These conditions could result in this community phase reaching a threshold (T2A) where it becomes a noxious weed-dominated state.
Restoration pathway R3A
State 3 to 2
Although there are no known restorations efforts completed on this ecological site, studies indicate restoration may be expensive to implement and is generally reserved for the most productive land with easy access. Use of an integrated approach for control of noxious species is the most effective way to prevent one species from dominating and to improve range forage and productivity. Medusahead density may be controlled through a variety of measures, including grazing, mowing, burning and chemical treatments. Targeting the treatment to the plant life stage is an important factor in the reduction of invasive species (Di’Tomaso et al., 2007). Control activities are most effective in late spring; if the seed and foliage of medusahead is removed or killed before seeds mature, it does not have resources to recover (Kyser et al., 2014). Thatch control via grazing, mechanical means or burning is also important to assist in germination of desirable species. When utilizing prescribed burning careful timing is essential as burning following seed dispersal is largely ineffectual (DiTomaso and Johnson (eds.) 2006); burning before seed dehiscence is one of the most effective controls, with emphasis on exposing inflorescences to direct flame (Kyser et al., 2014). Caution should be exercised when utilizing prescribed fire, as it will also scarify yellow star-thistle seeds and enhance their germination. A chemical treatment is necessary as a follow-up treatment to burning if yellow star-thistle is present (pers. com., Gustafson). A comprehensive review of the use of fire and other control methods of medusahead has been prepared by Kyser and others (2014). Timing of control should closely follow flower initiation to prevent seed production (DiTomaso et al., 2006). Controlled grazing and the application of herbicides in a variety of studies acted to reduce populations of yellow star-thistle (DiTomaso et al., 2007). Chemical treatment early in the season was more effective in its control. Repeated burning has reduced the incidence of yellow star thistle in other studies. Several consecutive years of burning are required for control of yellow star-thistle (DiTomaso and Johnson (eds.), 2006). Burning should be utilized the first and possibly the second year with follow-up chemical treatment for best control (DiTomaso et al., 2007). Increasing the density of competing grasses or legumes through planting in combination with fertilization could aid in the control of this species (California Rangelands, UCD). A combination of weed control techniques can be effective in control of yellow star-thistle; several years are required to deplete the seed bank and establish competitive vegetation with subsequent monitoring to prevent re-infestation (DiTomaso et al., 2007). Revegetation with native species, especially perennials has been successful in some areas. Low nitrogen levels limit medusahead growth, and may allow for improved growth of perennials (Kyser et al., 2014). Reseeding with natives is expensive and is mostly reserved for the most productive lands.
Additional community tables
Table 9. Community 1.1 plant community composition
Group | Common name | Symbol | Scientific name | Annual production (kg/hectare) | Foliar cover (%) | |
---|---|---|---|---|---|---|
Grass/Grasslike
|
||||||
1 | Annual Grasses | 448–1177 | ||||
wild oat | AVFA | Avena fatua | 448–1121 | 22–52 | ||
desert fescue | VUMIM | Vulpia microstachys var. microstachys | 0–56 | 0–1 | ||
Forb
|
||||||
2 | Annual Forbs | 0–426 | ||||
knotted hedgeparsley | TONO | Torilis nodosa | 0–303 | 0–31 | ||
miner's lettuce | CLPE | Claytonia perfoliata | 0–78 | 0–7 | ||
common chickweed | STME2 | Stellaria media | 0–22 | 0–2 | ||
longbeak stork's bill | ERBO | Erodium botrys | 0–22 | 0–1 | ||
4 | Perennial forbs | 0–78 | ||||
Ithuriel's spear | TRLA16 | Triteleia laxa | 0–62 | 0–4 | ||
miniature lupine | LUBI | Lupinus bicolor | 0–17 | 0–2 | ||
Tree
|
||||||
3 | Trees | 717–852 | ||||
blue oak | QUDO | Quercus douglasii | 717–852 | 35–36 |
Table 10. Community 1.2 plant community composition
Group | Common name | Symbol | Scientific name | Annual production (kg/hectare) | Foliar cover (%) | |
---|---|---|---|---|---|---|
Forb
|
||||||
1 | Annual Forbs | 6–78 | ||||
dovefoot geranium | GEMO | Geranium molle | 0–34 | 0–1 | ||
little bur-clover | MEMI | Medicago minima | 0–22 | 0–1 | ||
knotted hedgeparsley | TONO | Torilis nodosa | 6–22 | 0–1 | ||
5 | Perennial Forbs | 0–67 | ||||
straightbeak buttercup | RAOR3 | Ranunculus orthorhynchus | 0–56 | 0–4 | ||
onion | ALLIU | Allium | 0–6 | 0–1 | ||
bluedicks | DICA14 | Dichelostemma capitatum | 0–6 | 0–1 | ||
Grass/Grasslike
|
||||||
2 | Grasses | 639–1311 | ||||
wild oat | AVFA | Avena fatua | 639–1211 | 40–60 | ||
perennial ryegrass | LOPE | Lolium perenne | 0–67 | 0–1 | ||
soft brome | BRHO2 | Bromus hordeaceus | 0–17 | 0–1 | ||
compact brome | BRMA3 | Bromus madritensis | 0–11 | 0–1 | ||
red brome | BRRU2 | Bromus rubens | 0–6 | 0–1 | ||
Shrub/Vine
|
||||||
3 | Shrubs | 34–151 | ||||
whiteleaf manzanita | ARMA | Arctostaphylos manzanita | 34–135 | 2–5 | ||
birchleaf mountain mahogany | CEMOG | Cercocarpus montanus var. glaber | 0–11 | 0–4 | ||
scrub oak | QUBE5 | Quercus berberidifolia | 0–6 | 0–3 | ||
Tree
|
||||||
4 | Trees | 146–448 | ||||
blue oak | QUDO | Quercus douglasii | 146–448 | 15–20 | ||
California foothill pine | PISA2 | Pinus sabiniana | 0–11 | 0–2 |
Interpretations
Animal community
Wildlife
Oak savannas and woodlands may provide essential habitat elements for a variety of wildlife species. Due to the natural mosaic of grassland, shrubs and trees, a variety of micro-habitats are provided, meeting some of the reproductive, foraging and/or cover requirements for wildlife. In one study in central California, habitat elements that included shrubs, grass and down wood were positively associated with the abundance of small mammals, and shrub cover and litter weight with abundance of birds and reptiles (Tieje et al., 1997).
Of the 632 terrestrial vertebrates (amphibians, reptiles, birds, and mammals) native to California, over 300 species use oak woodlands for food, cover and reproduction, including at least 120 species of mammals, 147 species of birds and approximately 60 species of amphibians and reptiles (Tietje et al., 1997). Common species on this site include Beechey ground squirrels (Spermophilus beecheyi), Botta pocket gopher (Thomomys bottae mewa), and Audubon cottontail (Sylvilagus audubonii vallicola). The rich rodent and rabbit population is an important food source for common predators including: bobcat (Lynx rufus californicus), coyote (Canis latrans) and the Pacific rattlesnake (Crotalus viridis oreganus).
Other wildlife species found in oak savannas include several important game animals, such as Columbian blacktail deer (Odocoileus hemionus columbiana), California quail (Callipepla californica), and the "re-introduced" wild turkey (Meleagris gallopavo) that contribute to California's economy through revenues from recreational hunting (Garrison and Standiford, 1997).
Birds can serve as “focal species” in that their requirements define spatial attributes, habitat characteristics and management regimes for a healthy system (Zack, 2002). Bird species have essential habitat elements that include large oak trees with associated cavities and acorns, snags, shrubs, grasses and forbs, brush piles and water. Oak woodlands are important over-wintering environments for large numbers of Neotropical migratory birds such as flycatchers, vireos, and warblers. Acorn woodpeckers (Melanerpes formicivoris) and western scrub jays (Aphelocoma californica) forage heavily on acorns, and oak titmice (Baeolophus inornatus), western bluebirds (Sialia Mexicana) and tree swallows (Tachycineta bicolor) nest in the cavities of oaks. Many types of eagles, kites, hawks and owls use oak savannas for breeding and the abundance of prey found on the landscape. Important habitat elements include snags, granaries, woody debris, shrubs, and brushpiles (Garrison and Standiford, 1997).
Community phase 1.2 has the important shrub structure required by many birds. Brush provides wildlife habitat in the form of good hiding and fawning cover for deer and forage and hiding places for birds. Birds perch in the trees and contribute to shrub dispersal (Block, 1990). Forage and cover available for birds and other animals on these sites is low to moderate. Habitat use should be a consideration in management alternatives.
The numerous diseases that affect the heartwood of oak boles and limbs create important cavities for a variety of tree dwellers. Mistletoe in oaks (Phorodendron villosum) has berries that are attractive to birds that eat and excrete the live seeds which then stick and create a new plant (Perry and Elmore, 2006).
Grazing and Browsing
The primary limitation for livestock production on this site is lack of water sources during most of the year. Range forage is optimal for livestock growth and production for only a short period of the year (George, 1993). The quality of range forage varies with plant species, season, location, and range improvement practices.
The browse value of common oak woodland species is listed in Sampson and Jespersen (1963). Yellow star-thistle is an important plant for the honey bee industry as it provides late season food source for bees (DiTomaso, 2006). Cattle may graze on it early in the season up to the bolting stage but thereafter it is unpalatable to most livestock (DiTomaso, 2006). It is toxic to horses.
Acorns are eaten by at least a dozen species of songbirds, several upland game birds, rodents, black-tailed deer, feral and domestic pig, and all other classes of livestock (Adams et al, 1992; Duncan and Clawson 1980; Sampson and Jespersen 1963). Acorns are a critical food source for deer, which migrate from high-elevation dry summer ranges to blue oak woodland for fall and winter forage (Burns and Honkala 1990). Deer, rodents and rabbits browse blue oak and contribute to poor survival of oak natural regeneration.
Manzanita berries are utilized by a number of birds and small animals (Stuart and Sawyer, 2001). Young oak seedlings leaves are browsed by deer and cattle.
Hydrological functions
The watersheds associated with these sites are drained by intermittent streams that only flow during the wet season. In dry years these intermittent streams may not flow at all. Runoff on these soils is rapid and soil erosion hazard is high.
Recreational uses
Bird watching, hunting, camping, horseback riding, all-terrain vehicle riding, and hiking in spring and near developed reservoirs are common recreational pursuits.
Wood products
Blue oak is often utilized for firewood.
Other products
Native Americans have historically used and managed the blue oak savanna and woodlands for food and fiber. The gathering of native plants such as bulbs and corms, grasses and brush for food, medicine and crafts is still practiced today (Anderson, 2005). Historically these gathering methods sustained local plant populations and promoted plant diversity.
Other information
General
Soils:
The capacity of the soil to function is affected by dynamic soil properties (Tugel and Brown, 2001) that describe soil resistance and resilience. “Dynamic properties include organic matter, soil structure, infiltration rate, bulk density, and water and nutrient holding capacity” (Soil Quality.Org, 2012). Soil resistance is defined as: "the capacity of a soil to continue to function without change throughout a disturbance" and soil resilience is defined as "the capacity of a soil to recover its functional and structural integrity after a disturbance" (Seybold et al., 1999). “Changes in those properties depend both on land management practices and the inherent properties of the soil” (Soil Quality.Org, 2012).
These soils have some properties that have a high resistance to disturbance; for example they have a high volume (moderate to deep depth) to absorb and buffer compaction.
When these soils are wet they are “plastic” or susceptible to deformation under stress and have a low resistance to disturbance (Virmani, Sarawat and Burford, 1982). Slow permeability and steep slopes also make this site susceptible to erosion if disturbed. This soil has a high resilience when dry with some ability to recover when disturbed.
Revegetation/Restoration of Disturbed Areas
Native Grass Restoration:
Native perennial grasses may occur on this ecological site in very small amounts. There is no known practice or group of practices that can successfully restore native grasses on this ecological site.
Poisonous/Non-native Plants
Poisonous Plants:
There are several poisonous plants on this ecological site. Pyrrolizidine alkaloids in fiddleneck (Amsinkia spp.) can cause liver damage in livestock. Acorns and oak leaves eaten in excess (up to 50 percent of the diet) may be toxic to cattle (Sullins and Maas, 2011). Heavy acorn years in combination with early spring rains and/or snow may cause cattle to consume oak forage resulting in toxicity and possible death. Immediate supplemental feeding of hay is recommended as well as movement away from the area to avoid cattle deaths from oak toxicity (Sullins and Maas, 2011). Livestock poisoning is a result of hungry animals being concentrated on toxic plants (George, R015XI002, August 2007).
Invasive Species:
Medusahead (Taeniatherum caput-medusae) and yellow starthistle (Centaurea solstitalis) may invade this ecological site.
Supporting information
Inventory data references
Information utilized to develop the Ecological Site Concept and plant communities includes the following:
2 Range 417s from 1980 and 1992
ES Inventory Plot Data:
10 step point transects, 6 line intercept transects,7 production (double sampling) plots
Type locality
Location 1: Colusa County, CA | |
---|---|
Township/Range/Section | T18N R5W S34 |
UTM zone | N |
UTM northing | 0551330 |
UTM easting | 4357802 |
General legal description | Off Sites Lodoga road about 1/2 mile, midpoint on northwest facing slope. |
Other references
Abrahamson, I. 2014. Arctostaphylos manzanita. In: Fire Effects Information System, [Online]. U.S. Dep. of Agric., For. Serv., Rocky Mountain Res. Sta., Fire Sci. Lab. (Producer). Available: http://www.fs.fed.us/database/feis/ [2014, July 21].
Adams, T. E., P. B. Sands, W.H. Weitkamp, and N. K. McDougald. 1992. Oak seedling establishment on California rangelands. J. Range Manage. 45: 93-98.
Allen-Diaz, B., R. R. Evett, B. A. Holzman, and A. J. Martin. 1989. Report on Rangeland Cover Type Descriptions for California Hardwood Rangelands. Forest and Rangeland Resources Assessment Program, Calif. Dep. of Forestry and Fire Protection, Sacramento, Calif. 318 pgs.
Anderson, M.K. 2005. Tending the Wild, Native American Knowledge and the Management of California’s Natural Resources. University of California Press.
Arno, S. H. and S. Allison-Bunnell. 2002. Flames in Our Forest. Island Press. 227 pgs.
Baker, H.G. Sources of the naturalized grasses and herbs in California. In: Huenneke, L.F. and H.A. Mooney (ed.). 1989. Grassland Structure and Function: California Annual Grassland. Kluwer Academic Publishers, Dordrecht, Netherlands. Pg. 29-38.
Baldocchi, D.D., and L. Xu. 2007. What limits evaporation from Mediterranean oak woodlands – The supply of moisture in the soil, physiological control or the demand by the atmosphere? Elsevier Ltd. Doi:10.1016/j.advwatres.2006.06.013. Advances in Water Resources. 30 (2007) 2113-2122. www.elsevier.com/locate/advwatres
Baldocchi, D.D., Xu, L., and N. Kiang. 2004. How plant functional type, seasonal drought and soil physical properties alter water and energy fluxes on oak-grass savanna and an annual grassland. Agr. and For. Meterology. Vol.123, issues 1-2, pg.13-39.
Barbour, M.G., and J. Major. Terrestrial Vegetation of California. 1977. John Wiley and Sons, Inc.
Bartolome, J. W. 1987. California grassland and oak savannah. Rangelands 9:122-125.
Bartolome, J.W., W.F. Frost, N.K. McDougald and M. Connor. 2002. California guidelines for residual dry matter (RDM) management on coastal and foothill annual rangelands. Rangeland Monitoring Series. Publ. 8092, Div. of Agr. and Nat Res., Univ. of Calif. 8pp.
Blackburn, T.C. and K. Anderson. 1993. Before The Wilderness: Environmental Management By Native Californians. Ballena Press, Menlo Park, CA.
Bolsinger, C. L. 1988. The hardwoods of California’s timberlands, woodlands, and savannas. Res. Bull. PNW-RB-148. Portland, OR: U.S. Department of Agriculture, Forest Service, Pacific Northwest Research Station. 148 p.
Burcham, L. T. 1957. California Rangeland. Div. Forestry, Sacramento, Calif. 261 pgs.
Burns, R. M. and B. H. Honkala. (eds.) 1990. Silvics of North America (Vol. 2): Hardwoods. Agric. Handbook 654. USDA Forest. Service, Washington D.C. 877 p.
Caldwell, M.M., Dawson, T.E., and J.R. Richards Hydraulic lift: consequences of water efflux from the roots of plants. 1998. Oecologia 113:151-161.
Conner, M. 1991. http://ucanr.edu/sites/oak_range/Oak_Articles_On_Line/Factors Affecting Annual Forage Yield in Oak Woodlands. Accessed 2015.
Conner, M. 1996
http://ucanr.edu/sites/oak_range/Oak_Articles_On_Line/Oak_Woodland_Products_Range_Management_Livestock/ Accessed 2015.
Dahlgren R, Horwath W, Tate K, Camping T. 2003. Blue oak enhance soil quality in California oak woodlands. Calif Agr 57(2):42-47. DOI: 10.3733/ca.v057n02p42
Dahlgren, R.A., K.W. Tate, D. Lewis, D.R. Atwill, J.M. Harper, and B. Allen-Diaz. 2001. Watershed research examines rangeland management effects on water quality. CALIFORNIA AGRICULTURE, VOL. 55, No. 6.
Daniel, J.A., K. Potter, W. Altom, H. Aljoe, and R. Stevens. 2002. Long-Term Grazing Density Impacts on Soil Compaction. Transactions of the ASAE, 45:1911-1915.
Daryanto, S. and D.J. Eldridge. 2010. Shrub removal and grazing alter the spatial distribution of infiltrability in a shrub-encroached woodland. In: Proceedings of the 16th Biennial Conference of the Australian Rangeland Society, Bourke (Eds. D.J. Eldridge and C. Waters) (Australian Rangeland Society:Perth)
D’Antonio, C.D, S. Bainbridge, C. Kennedy, J. Bartolome, S. Reynold. 2006. Ecology and Restoration of California Grasslands with special emphasis on the influence of fire and grazing on native grassland species. University of California, Berkeley. Department of Integrative Biology.
DiTomaso, J.M., G.B. Kyser, W.T. Lanini, C.D. Thomsen, T.S. Prather. 2007. Yellow Star-Thistle. July 28, 2014. http://www.ipm.ucdavis.edu/PMG/PESTNOTES/pn7402.html
DiTomaso, J.M., G.B. Kyser, and M.J. Pitcairn. 2006. Yellow Starthistle Management Guide. California Invasive Pest Council. http://www.cal ipc.org/ip/management/plant_profiles/Centaurea_solstitialis.php
Duncan, D. A. and W.J. Clawson. 1980. Livestock utilization of California’s oak woodlands. In: Plumb, Timothy R., (technical coordinator). Proceedings of the symposium on the ecology, management, and utilization of California oaks. Gen. Tech. Rep. PSW-44. U.S. Dep. of Agr., For. Serv. Pacific Southwest Forest and Range Exp. Sta., Berkeley, CA. Pgs. 306-313.
Eviner, V.T. and F.S. Chapin III, 2001. Plant species provide vital ecosystem functions for sustainable agriculture, rangeland management and restoration. California Agriculture, Volume 55, Number 6. Pgs. 54-59.
Fryer, J. L. 2007. Quercus douglasii. In: Fire Effects Information System, [Online]. U.S. Department of Agriculture, Forest Service, Rocky Mountain Research Station, Fire Sciences Laboratory (Producer). Available: http://www.fs.fed.us/database/feis/ [2013, February 19].
Garrison, B.A., and R.B. Standiford, 1997. A Post-Hoc Assessment of the Impacts to Wildlife Habitat from Wood Cutting in Blue Oak Woodlands in the Northern Sacramento Valley. USDA Forest Service Gen. Tech. Rep. PSW-GTR-160.
George, M., Harper, J., Davy, J., and T. Bechetti. Livestock Production. http://californiarangeland.ucdavis.edu/Livestock_Production/ accessed May 1, 2015.
George, M., J. Clawson, J. Menke, and J. Bartolome. 1985. Annual grassland Forage Productivity. 1985. In: Rangelands 7(1), 3 pgs.
George, M., J. Bartolome, N. McDougald, M. Connor, C. Vaughn and G. Markegard. 2001a. Annual Range Forage Production. ANR Publ. 8018, Div. of Agric. And Nat. Res., Univ. of Calif., Oakland, Calif. 9 pgs.
George, M., G. Nader, N. McDougald, M. Connor, and B. Frost. 2001b. Annual Rangeland Forage Quality. ANR Publ. 8022, Div. of Agric. And Nat. Res., Univ. of Calif., Oakland, Calif. 13 pgs.
George, M.R., J.R. Brown and W.J. Clawson. 1992. Application of non-equilibrium ecology to management of Mediterranean grasslands. J. Range Manage. 45: 436-440.
George, M. 1993. ESDs https://esis.sc.egov.usda.gov/
Griffin, D., and K. J. Anchukaitis (2014), How unusual is the 2012–2014 California drought?, Geophys. Res. Lett., 41, 9017–9023, doi:10.1002/2014GL062433.
Grünzweig, J.M., Carmel, Y., Riov, J., Sever, N., McCreary, D.D., and C.H. Flather, 2008. Growth, resource storage, and adaptation to drought in California and eastern Mediterranean oak seedlings. Can. J. For. 38: 331-342 (2008) doi:10.1139/X07-152
Hahn, G.L. 1995. Weather and Climate Impacts to Beef Cattle. USDA, Ag. Res. Sta., Lincoln, Nebraska. Published in Beef Research Program Progress Report (1985) No. 2: 85-89
Harper, J.M., Standiford, R.B., and J. Leblanc. 1991. http://ucanr.edu/sites/oak-range/Oak_Articles_On_Line/Oaks and Climatic Factors/The Effect of Drought on California Oaks.
Heady, H. F. 1956. Changes in a California Annual Plant Community Induced by Manipulation of Natural Mulch. In Ecology, Vol.7, No.4. pgs. 798-812.
Herman, D.J., Halverson, L.J., and M.K. Firestone, 2003. Nitrogen Dynamics in an Annual Grassland: Oak Canopy, Climate and Microbial Population Effects. Ecological applications, 13(3) 2003, pp. 593-604.
Hickman, G.W., E.J. Perry, and R.M. Davis. 2011. Wood Decay Fungi in Landscape Trees. University of California. Integrated Pest Management Program. Agriculture and Natural Resources. Pest Notes 74109.
Holloran, P., Mackenzie, A., Farrell, S., Johnson, D., 2004. A Guide to Techniques for Removing Bay Area Invasive Plants. The Watershed and Invasive Plant Council. 128 pgs.
Ishikawa, C.M. and C.S. Bledsoe. 2000. Seasonal and diurnal patterns of soil water potential in the rhizosphere of blue oaks: evidence of hydraulic lift. Oecologia 125:459-465. DOI 10.1007/s004420000470
Jetter, K.M., J.M. DiTomaso, D.J. Drake, K.M. Klonsky, M.J. Pitcairn, and D.A. Sumner. 2003. "Biological Control of Yellow Starthistle." Exotic Pests and Diseases: Biology and Economics for Biosecurity, Daniel A. Sumner, ed., pp.225-241. Ames: Iowa State University Press.
Kertis, J.A., R. Gross, D.L. Peterson, M.J. Arbaugh, R.B. Standiford, D.D. McCreary.1993. Growth trends of Blue oak (Quercus douglasii in California. Can. Jor. For. Res. 23:1720-1724.
Kreitlow, K.W. and R.H. Hart.1974. Plant morphogenesis as the basis for scientific management of range resources: Agricultural Research Service, U.S. Dept. of Agriculture. Proceedings of the workshop of the United States-Australia Rangelands Panel, Berkeley, California, March 29-April 5, 1971 (Google eBook)
Kueppers, L.M., M.A. Synder, L.C. Sloan, E.S. Zavaleta, B. Fulfrost. 2005. Modeled regional climate change and endemic oak ranges. Proceedings of the National Academy of Sciences of the United States of America. www.pnas.org_cgi_doi_10.1073_pnas.0501427102. Vol. 102. No.45.
Kyser, G.B., DiTomaso, J.M., Davies, K.W., Davy, J.S, and B.S. Smith (2014). Medusahead Management Guide for the Western States. Univ. of Calif.
Liste, H-H., and J.C. White, 2008. Plant hydraulic lift of soil water- implications for crop production and land restoration. Plant Soil (2008) 313:1-17 DOI 10.1007/s11104-008-9696z
Mayer K. E., and W. F. Laudenslayer. (Eds.) 1988. A guide to wildlife habitats of California. California Dept. of Forestry and Fire Protection, Sacramento.
McCreary, D.D. and M. George. 2005. Managed Grazing and Seedling Shelters Enhance oak Regeneration on Rangelands.
http://californiaagriculture.ucop.edu/
McCreary, D.D., Tietje, W., and W. Frost. 2008. Stump Sprouting 19 Years after Harvest. Gen. Tech. Rep. PSW-GTR-217.
McCreary, D.D. Fire in California’s Oak Woodlands. 2004. http://danr.ucop.edu/ihrmp
McCreary, D.D., Tietje, W.D., and W. Frost. 2002. Stump sprouting of Blue Oaks Ten Years after Harvest. USDA For. Serv., Gen.Tech.Rep. PSW-GTR-184. 8 pgs.
McCreary, D. D. 2001. Regenerating rangeland oaks in California. ANR Publ. 21601, Div. of Agric. And Nat. Res., Univ. of Calif., Oakland, Calif. 62 pgs.
McClaran, M.P. 1986. Age structure of Quercus douglasii in relation to livestock grazing and fire. Ph.D. Dissertation. Univ. of Calif., Berkeley. 119 pp.
McDougald, N.K. W.E. Frost, and W.J. Clawson. 1991. Estimating the cost of replacing forage losses on annual rangeland. Leaflet 21494. Division of Agric. and Nat. Res., Univ. of Calif., Oakland, Calif.
McDonald, P. M. 1990. Quercus douglasii Hook & Arn. Blue oak. In: Burns, Russell M.; Honkala, Barbara H., tech. coords. Silvics of North America. Vol.2. Hardwoods. Agric. Handb. 654. Washington, DC: U.S. Department of Agriculture, Forest Service: 631-639.
Mensing, S. A. 1992. The impact of European settlement on blue oak (Quercus douglasii) regeneration and recruitment in the Tehachapi Mountains, California. Madrono. 39: 36-46.
Miller, G.R., Chen, X., Rubin, Y., Ma, S., and D.D. Baldocchi. 2010. Ground water uptake by woody plants in a semi-arid oak savanna. Water Resources Research, Vol. 46, W10503, http://dx.doi.org/10.1029/2009WR008902
Miller, G.R., Cable, J.M., McDonald, A.K., Bond, B., Franz, T.E., Wang, L., Gou, S., Tyler, A.P., Zou, C.B., and R.L. Scott. 2012. Understanding ecological connectivity in savannas: A system dynamics modelling approach. Ecohydrology. 5,200-220. Published Online 7 July 2011 in Wiley Online Library (wileyonlinelibrary.com) DOI: 10.1002/eco.245
O’Geen. A.T., Dalgren, R.A., Swarowsky, A., Tate, K.W., Lewis, D.J., and M.J. Singer. 2010. Research connects soil hydrology and stream water chemistry in California oak woodlands. CA. Agric. Vol. 64. No.2. http://escholarship.org/uc/item/34x9m71j
Parsons, D.J and T.K. Stohlgren. 1989. Effects of varying fire regimes on annual grasslands in the southern Sierra Nevada of California. Madroño, 36:154-168.
Pavlik, B.M., P.C. Muick, S. Johnson, and M. Popper. 1991. Oaks of California. Cachuma Press, Inc. Los Olivos, Calif. 184 pgs.
Perry, E.J. and C.L Elmore. 2006. University of California. Mistletoe. Integrated Pest Management Program. Agriculture and Natural Resources. Pest Notes 7437.
Philips, R.L., N.K. McDougald, D. McCreary, and E.R. Atwill. 2007. Blue oak seedling age influences growth and mortality. California Agriculture, Vol.61, No.1.
Philips, R.L., N.K. McDougald, E.R. Atwill, and D. McCreary. 2007. Exclosure size affects young blue oak seedling size. California Agriculture, Vol.61, No.1. 4pgs.
Pickett, S.T.A., and P.S. White. 1985. The Ecology of Natural Disturbance and Patch Dynamics. Academic Press. 472 Pgs.
Purcell, K.L., Stephens, S.L., 2005. Changing fire regimes and the avifauna of
California oak woodlands. Studies in Avian Biology 30, 33–45.
Quinn, R.D. and S.C. Keeley. 2006. Introduction to California Chapparal. University of California Press. 322 pgs.
Rice, K.J. and E.K. Espeland. 2007. Genes on the range: Population genetics. In: Ecology and
Conservation of California Grasslands. J. Corbin, C. D’Antonio, M. Stromberg eds. University of California Press, Berkeley.
Sampson, A. W. and B. S. Jespersen. 1963. California range brushlands and browse plants. Univ. of Calif. Div. of Agr. Sci., Berkeley, CA. 162 pgs.
Sawyer, J. O., Keeler-Wolf, T., and J. M. Evens. 2009. A Manual of California Vegetation, Second Edition. California Native Plant Society Press. Sacramento, CA.
Stahle D.W., R.D. Griffin, D.M. Meko, M.D. Therrel, J.R. Edmondson, M.K. Cleaveland, L.N. Stahle, D.J. Burnette, J.T. Abatzoglou , K.T. Redmond, M.D. Dettinger, and D.R Cayan. 2013. The Ancient Blue Oak Woodlands of California: Longevity and Hydroclimatic History. Earth Interactions Volume 17. Paper No. 12.
Standiford, R.B., McCreary, D.D., Barry, S.J., Forero, L.C., 2011. Blue oak stump sprouting evaluated after firewood harvest in northern Sacramento Valley. Ca. Agric. Vol. 65, No. 3. http:/scholarship.org/us/item/5pv6j50d
Stephens, S.L. Fire history of mixed oak-pine forest in the foothills of the Sierra Nevada, El Dorado County, Calif. In: N.H. Pillsbury, J. Verner, and W.D. Tietje (Ed). 1997. Proceedings, Symposium on Oak Woodlands: Ecology, Management, and Urban Interface Issues. USDA Forest Service GTR-PSW GTR-160.
Stromberg, M.R., J.D. Corbin, C.M. D’Antonio. 2007. California Grasslands: Ecology and Management. University of California Press, Berkeley and Los Angeles, CA. 390 pgs.
Stuart, J.D. and J.O. Sawyer, 2001. Trees and Shrubs of California. University of California Press. 467 pgs.
Sullins, J. and J. Maas. 2011. Blue oak Acorn Toxicity Risk in Cattle.
http://ucanr.edu/blogs/blogcore/
Sweitzer, Rick A. and Dirk H. Van Vuren. 2002. Rooting and Foraging Effects of Wild Pigs on Tree Regeneration and Oak Survival in California’s Oak Woodland Ecosystems. USDA Forest Service Gen. Tech. Rep. PSW-GTR-184.
Tietje, W.D., J.K. Vreeland, N.R. Siepal, and J.L. Dockter. 1997. Relative Abundance and Habitat Associations of Vertebrates in Oak Woodlands in Coastal Central California. USDA Forest Service Gen. Tech. Rep. PSW-GTR-160. 1997.
University of California Agriculture ~ California Agriculture ~ January-March 2007 http://calag.ucop.edu/0701JFM/resup02.html
University of California. UC Davis. California rangelands. http://californiarangeland.ucdavis.edu/Weeds/ Accessed May 2014.
University of California Agriculture ~ California Agriculture ~ January-March 2007. Oaks Research Update. http://calag.ucop.edu/0701JFM/resup02.html
University of California. 2010. Sudden Oak Death. UC Statewide Integrated Pest Management Program. Agriculture and Natural Resources. http://www.ipm.ucdavis.edu/PMG/PESTNOTES/pn74151.html
Vayssieres M.P. and R.E. Plant. 1998. Identification of vegetation state-and-transition domains in California's hardwood rangelands. FRAP Publication, California Department of Forestry and Fire Protection. Sacramento, CA.
Virmani, S.M., K.L. Sahrawat, and J.R. Burford. 1982. Physical and Chemical Properties of Vertisols and Their Management. International Crops Research Institute for the Semi-Arid Tropics (ICRISAT), Patancheru, Andhra Pradesh, India.
Young, J.A., Evans, R.A., Raguse, C.A., and J. R. Larson, 1981. Germinable seeds and periodicity of germination in annual grasslands. Hilgardia 49:1-37.
Zack, S. 2002. Oak Woodland Bird Conservation Plan, A Strategy for Protecting and Managing Oak Woodland Habitats and Associated Birds in California. California Partners in Flight. V.2.0
Contributors
Judith Welles
Rangeland health reference sheet
Interpreting Indicators of Rangeland Health is a qualitative assessment protocol used to determine ecosystem condition based on benchmark characteristics described in the Reference Sheet. A suite of 17 (or more) indicators are typically considered in an assessment. The ecological site(s) representative of an assessment location must be known prior to applying the protocol and must be verified based on soils and climate. Current plant community cannot be used to identify the ecological site.
Author(s)/participant(s) | Judy Welles, Ryan Miebach |
---|---|
Contact for lead author | Chico Soil Survey Office, Chico, CA |
Date | 09/19/2013 |
Approved by | |
Approval date | |
Composition (Indicators 10 and 12) based on | Annual Production |
Indicators
-
Number and extent of rills:
Some rilling would be expected. After heavy spring rains rilling (2 rills per 20 feet) was noted. -
Presence of water flow patterns:
Water commonly flows downslope for a length of 200-500 feet. -
Number and height of erosional pedestals or terracettes:
No erosion pedestals were noted. Some terracettes would be expected. -
Bare ground from Ecological Site Description or other studies (rock, litter, lichen, moss, plant canopy are not bare ground):
Bare ground ranges from 10 to 40 percent. -
Number of gullies and erosion associated with gullies:
These soils may be found in association with gullies that are 4 to 6 feet deep at 500 to 1000 foot intervals. -
Extent of wind scoured, blowouts and/or depositional areas:
No wind scour or blowouts were noted. -
Amount of litter movement (describe size and distance expected to travel):
Very little if any litter movement was noted. Oak leaves would be 2-3 inches long and 1-2 inches wide. Grass litter would be 4-6 inches long and 1/10 inches wide, and forbs would be2-3 inches long and 1/2 inch wide. -
Soil surface (top few mm) resistance to erosion (stability values are averages - most sites will show a range of values):
Soil surface is clay loam, silty clay, silty clay loam and clay with a medium blocky structure. When these soils are wet they are “plastic” or susceptible to deformation under stress and have a low resistance to disturbance (Virmani, Sarawat and Burford, 1982). This soil also has a high resilience when dry with some ability to recover when disturbed. Slow permeability and steep slopes also make this site susceptible to erosion if disturbed. Soil erosion hazard is slight to high. -
Soil surface structure and SOM content (include type of structure and A-horizon color and thickness):
Sehorn: A horizon is 0 to 7 inches; pale brown (10YR 6/3) clay loam, dark brown (10YR 4/3) with medium subangular blocky structure. SOM 1-4 percent.
Altamont: A--0 to 7 inches; brown (10YR 4/3) clay, dark brown (10YR 3/3) with strong coarse prismatic and strong coarse and medium blocky structure. SOM 1-4 percent. -
Effect of community phase composition (relative proportion of different functional groups) and spatial distribution on infiltration and runoff:
Grass>>>Trees>>Shrubs>Forbs
High grass cover on foot slopes should prevent soil loss from rainfall impact and grass cover slows runoff. Backslopes would have less grass cover. The presence of trees intercepts rainfall and stem flow and roots aid water infiltration. While shrubs aid infiltration, their low cover does not significantly contribute to overall infiltration and runoff. Forbs have little to no effect on infiltration and runoff
-
Presence and thickness of compaction layer (usually none; describe soil profile features which may be mistaken for compaction on this site):
None noted. Platy structure could be confuse with compaction. -
Functional/Structural Groups (list in order of descending dominance by above-ground annual-production or live foliar cover using symbols: >>, >, = to indicate much greater than, greater than, and equal to):
Dominant:
Annual grassesSub-dominant:
Blue oaksOther:
Common manzanitaAdditional:
-
Amount of plant mortality and decadence (include which functional groups are expected to show mortality or decadence):
Annual grasses and forbs will show mortality and decadence beginning in late April or early May. Blue oak would not be expected to have more than 1-2 percent mortality. -
Average percent litter cover (%) and depth ( in):
-
Expected annual annual-production (this is TOTAL above-ground annual-production, not just forage annual-production):
Expected production is highly variable based on unfavorable normal or favorable year. Total production ranges from approximately 750 to 1800 pounds per acre. -
Potential invasive (including noxious) species (native and non-native). List species which BOTH characterize degraded states and have the potential to become a dominant or co-dominant species on the ecological site if their future establishment and growth is not actively controlled by management interventions. Species that become dominant for only one to several years (e.g., short-term response to drought or wildfire) are not invasive plants. Note that unlike other indicators, we are describing what is NOT expected in the reference state for the ecological site:
Two common invasives could become an issue on footslope positions: Medusahead and
Yellow star-thistle
-
Perennial plant reproductive capability:
Minor amounts of native and non-native perennial grasses exist on the site including Melic spp. and Stipa spp.. Typically the native perennial grasses face strong competion from non-native grasses and forbs. Wet years with fall and winter rains tend to favor non-native grasses on well drained deep soils (Stromberg etal, 2007). Expected perennial grass seedlings would be 5 per 20 square foot.
Print Options
Sections
Font
Other
The Ecosystem Dynamics Interpretive Tool is an information system framework developed by the USDA-ARS Jornada Experimental Range, USDA Natural Resources Conservation Service, and New Mexico State University.
Click on box and path labels to scroll to the respective text.