
Natural Resources
Conservation Service
Ecological site F108XC530IA
Sandy Floodplain Forest
Last updated: 11/04/2024
Accessed: 04/13/2025
General information
Provisional. A provisional ecological site description has undergone quality control and quality assurance review. It contains a working state and transition model and enough information to identify the ecological site.
Figure 1. Mapped extent
Areas shown in blue indicate the maximum mapped extent of this ecological site. Other ecological sites likely occur within the highlighted areas. It is also possible for this ecological site to occur outside of highlighted areas if detailed soil survey has not been completed or recently updated.
MLRA notes
Major Land Resource Area (MLRA): 108X–Illinois and Iowa Deep Loess and Drift
The Illinois and Iowa Deep Loess and Drift, West-Central Part (MLRA 108C) encompasses the eastern portion of the Southern Iowa Drift Plain and the Lake Calvin basin of the Mississippi Alluvial Plain landforms (Prior 1991). It lies entirely in one state (Iowa), containing approximately 9,805 square miles (Figure 1). The elevation ranges from approximately 1,110 feet above sea level (ASL) on the highest ridges to about 505 feet ASL in the lowest valleys. Local elevation difference is mainly 10 to 20 feet. However, some valley floors can range from 80 to 200 feet, while some upland flats and valley floors only range between 3 and 6 feet. The MLRA is underlain by Pre-Illinoian glacial till, deposited more than 500,000 years ago and since undergone extensive erosion and dissection. In the northern half of the area the till thickness ranges from 150 to 350 feet and grades to less than 150 feet thick in the southern half. The till is covered by a mantle of Peoria Loess on the hillslopes and Holocene alluvium in the drainageways. Paleozoic bedrock, comprised of limestone, shale, and mudstones, lies beneath the glacial material (USDA-NRCS 2006).
The vegetation in the MLRA has undergone drastic changes over time. Spruce forests dominated the landscape 30,000 to 21,500 years ago. As the last glacial maximum peaked 21,500 to 16,000 years ago, they were replaced with open tundras and parklands. The end of the Pleistocene Epoch saw a warming climate that initially prompted the return of spruce forests, but as the warming continued, spruce trees were replaced by deciduous trees (Baker et al. 1990). Not until approximately 9,000 years ago did the vegetation transition to prairies as climatic conditions continued to warm and subsequently dry. Between 4,000 and 3,000 years ago, oak savannas began intermingling within the prairie landscape, while the more wooded and forested areas maintained a foothold in sheltered areas. This prairie-forest transition ecosystem formed the dominant landscapes until the arrival of European settlers (Baker et al. 1992).
Classification relationships
USFS Subregions: Central Dissected Till Plains (251C) Section, Central Dissected Till and Loess Plain (251Cc), Mississippi River and Illinois Alluvial Plains (51Cf), Southeast Iowa Rolling Loess Hills (251Ch) Subsections (Cleland et al. 2007)
U.S. EPA Level IV Ecoregion: Rolling Loess Prairies (47f), Upper Mississippi Alluvial Plain (72d) (USEPA 2013)
National Vegetation Classification – Ecological Systems: North-Central Interior Floodplain (CES202.694) (NatureServe 2015)
National Vegetation Classification - Plant Associations: Acer saccharinum – Fraxinus pensylvanica – Ulmus americana Floodplain Forest (CEGL002586) (Nature Serve 2015)
Biophysical Settings: Central Interior and Appalachian Floodplain Systems (BpS 4214710) (LANDFIRE 2009)
Natural Resources Conservation Service – Iowa Plant Community Species List: Forest, Silver Maple - Elm (USDA-NRCS 2007)
Iowa Department of Natural Resources: Floodplain Forest (INAI 1984)
U.S. Army Corps of Engineers: Floodplain Forests (Eggers and Reed 2015)
Ecological site concept
Sandy Floodplain Forests are located within the blue areas on the map (Figure 1). They occur on floodplains in river valleys. The soils are Entisols and Mollisols that are moderately well to excessively-drained and deep, formed in coarse-loamy alluvium. The site experiences seasonal flooding that can last up to 30 days.
The historic pre-European settlement vegetation on this ecological site was dominated by a dense, closed canopy of deciduous trees and a sparse understory of shade and flood-tolerant herbaceous plants. The tree canopy is comprised of silver maple (Acer saccharinum L.) and American elm (Ulmus americana L.). Other tree species that may occur can include eastern cottonwood (Populus deltoides W. Bartram ex Marshall), green ash (Fraxinus pensylvanica Marshall), slippery elm (Ulmus rubra Muhl.), boxelder (Acer negundo L.), and common hackberry (Celtis occidentalis L.) (NatureServe 2015). Vines are a common component and often include riverbank grape (Vitis riparia Michx.). The understory is populated with species tolerant of repeated flood disturbances with whitegrass (Leersia virginica Willd.) and Virginia wildrye (Elymus virginicus L.) the most commonly encountered. Shrubs are typically absent in late-successional communities, but early-successional communities may be dominated by black willow (Salix nigra L.). Seasonal flooding every one to two years is the primary disturbance factor that maintains this site (LANDFIRE 2009).
Associated sites
R108XC525IA |
Ponded Floodplain Marsh Mineral soils that are flooded and ponded including Aquents, Colo, Fluvaquents, Wabash, and Zook |
---|---|
R108XC526IA |
Floodplain Prairie Alluvial soils that are moderately well to well-drained, rarely to occasionally flooded, located furthest from the stream channel including Ankeny, Hanlon, Huntsville, and Kennebec |
R108XC527IA |
Wet Floodplain Sedge Meadow Alluvial soils that are poorly drained and rarely to occasionally flooded including Ambraw, Chequest, Coland, Colo, Dolbee, Elvira, Humeston, Ossian, Radford, Shaffton, Vesser, and Zook |
F108XC528IA |
Floodplain Swamp Forest Alluvial soils that are poorly drained and occasionally to frequently flooded including Colo and Quiver |
F108XC529IA |
Loamy Floodplain Forest Alluvial soils that are somewhat poorly to moderately well drained and occasionally flooded including Ackmore, Alluvial land, Amana, Arenzville, Lawson, Nodaway, Orion, and Spillville |
Similar sites
F108XC529IA |
Loamy Floodplain Forest Loamy Floodplain Forests are in a similar landscape position, but the fine-silty soils are somewhat poorly to moderately well-drained |
---|---|
F108XC528IA |
Floodplain Swamp Forest Floodplain Swamp Forests are in a similar landscape position, but the fine-silty soils are poorly-drained |
Table 1. Dominant plant species
Tree |
(1) Acer saccharinum |
---|---|
Shrub |
(1) Vitis riparia |
Herbaceous |
(1) Leersia virginica |
Physiographic features
Sandy Floodplain Forests occur on floodplains. They are situated on elevations ranging from approximately 400 to 1200 feet ASL. The site experiences occasional to frequent flooding that can last up to 30 days.
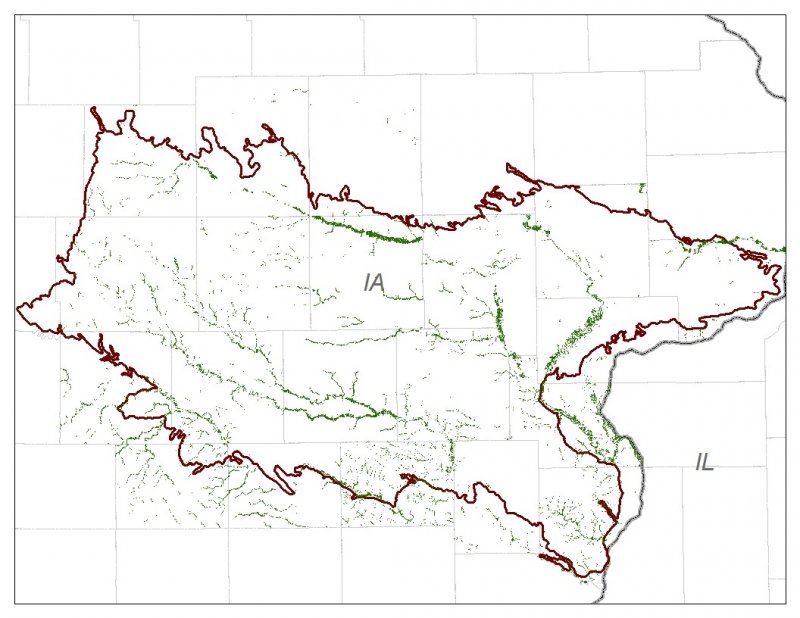
Figure 2. Figure 1. Location of Sandy Floodplain Forest ecological site within MLRA 108C.
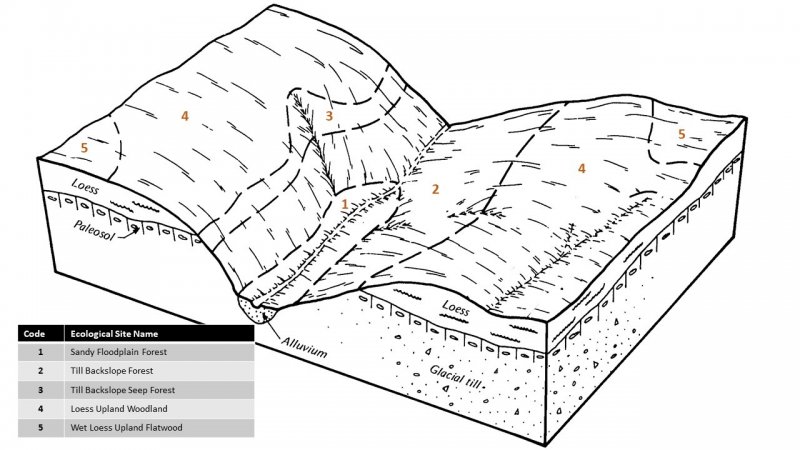
Figure 3. Figure 2. Representative block diagram of Sandy Floodplain Forest and associated ecological sites.
Table 2. Representative physiographic features
Slope shape across |
(1) Linear |
---|---|
Slope shape up-down |
(1) Convex (2) Linear |
Landforms |
(1)
River valley
> Flood plain
|
Runoff class | Negligible to very low |
Flooding duration | Brief (2 to 7 days) to long (7 to 30 days) |
Flooding frequency | Occasional to frequent |
Ponding frequency | None |
Elevation | 122 – 366 m |
Slope | 0 – 5% |
Water table depth | 122 – 203 cm |
Aspect | Aspect is not a significant factor |
Climatic features
The Illinois and Iowa Deep Loess and Drift, West-Central Part falls into the hot humid continental climate (Dfa) Köppen-Geiger climate classification (Peel et al. 2007). In winter, dry, cold air masses periodically shift south from Canada. As these air masses collide with humid air, snowfall and rainfall result. In summer, moist, warm air masses from the Gulf of Mexico migrate north, producing significant frontal or convective rains. Occasionally, hot, dry winds originating from the Desert Southwest will stagnate over the region, creating extended droughty periods in the summer from unusually high temperatures. Air masses from the Pacific Ocean can also spread into the region and dominate producing mild, dry weather in the autumn known as Indian Summers (NCDC 2006).
The soil temperature regime of MLRA 108C is classified as mesic, where the mean annual soil temperature is between 46 and 59°F (USDA-NRCS 2006). Temperature and precipitation occur along a north-south gradient, where temperature and precipitation increase the further south one travels. The average freeze-free period of this ecological site is about 178 days, while the frost-free period is about 157 days (Table 2). The majority of the precipitation occurs as rainfall in the form of convective thunderstorms during the growing season. Average annual precipitation is approximately 39 inches, which includes rainfall plus the water equivalent from snowfall (Table 3). The average annual low and high temperatures are 38 and 60°F, respectively.
Climate data and analyses are derived from 30-year averages gathered from four National Oceanic and Atmospheric Administration (NOAA) weather stations contained within the range of this ecological site (Table 4).
Table 3. Representative climatic features
Frost-free period (characteristic range) | 132-143 days |
---|---|
Freeze-free period (characteristic range) | 161-174 days |
Precipitation total (characteristic range) | 940-965 mm |
Frost-free period (actual range) | 132-148 days |
Freeze-free period (actual range) | 157-182 days |
Precipitation total (actual range) | 914-965 mm |
Frost-free period (average) | 138 days |
Freeze-free period (average) | 168 days |
Precipitation total (average) | 940 mm |
Figure 4. Monthly precipitation range
Figure 5. Monthly minimum temperature range
Figure 6. Monthly maximum temperature range
Figure 7. Monthly average minimum and maximum temperature
Figure 8. Annual precipitation pattern
Figure 9. Annual average temperature pattern
Climate stations used
-
(1) IOWA CITY [USC00134101], Iowa City, IA
-
(2) MARSHALLTOWN [USC00135198], Marshalltown, IA
-
(3) OSKALOOSA [USC00136327], Oskaloosa, IA
-
(4) BELLE PLAINE [USC00130600], Belle Plaine, IA
Influencing water features
Sandy Floodplain Forests are classified as a RIVERINE: Frequently Flooded; forested wetland under the Hydrogeomorphic (HGM) classification system (Smith et al. 1995; USDA-NRCS 2008) and as a Palustrine, Forested, Broad-leaved Deciduous, Temporarily Flooded wetland under the National Wetlands Inventory (FGDC 2013). Overbank flow from the channel and subsurface hydraulic connections are the main sources of water for this ecological site (Smith et al. 1995). Infiltration is moderate to high (Hydrologic Groups A and B) for undrained soils, and surface runoff is negligible to very low (Figure 5).
Primary wetland hydrology indicators for an intact Sandy Floodplain Forest may include: A1 Surface water, B1 Water marks, B2 Sediment deposits, B3 Drift deposits, and B9 Water-stained leaves. Secondary wetland hydrology indicators may include: D5 FAC-neutral test (USACE 2010).
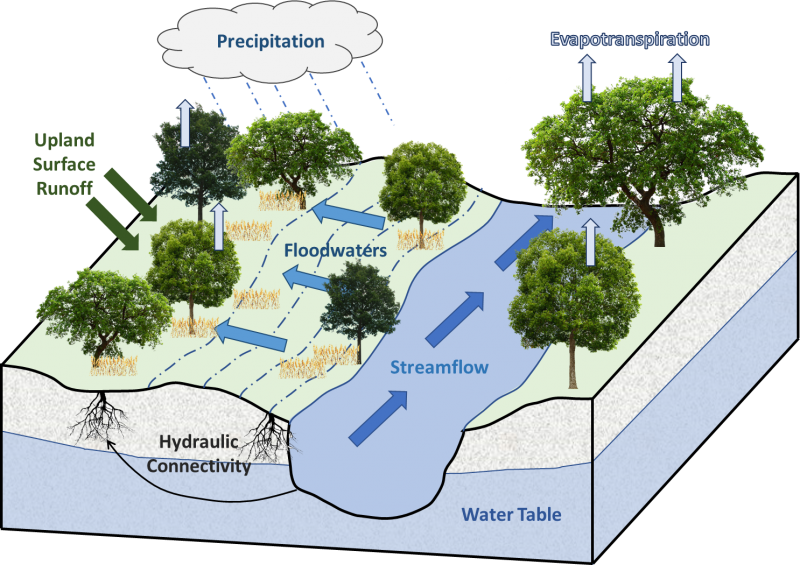
Figure 10. Figure 5. Hydrologic cycling in Sandy Floodplain Forest ecological site.
Soil features
Soils of Sandy Floodplain Forests are in the Entisols and Mollisols orders, further classified as Mollic Udifluvents, Typic Udifluvents, Typic Udipsamments, and Fluventic Hapludolls with moderate to High infiltration and negligible to very low runoff potential. While some of these soils are classified as Mollisols, their dark surfaces and increased thickness of the epipedon are not the result of prairie vegetation but rather alluvial deposition and slope wash. The soil series associated with this site includes Caneek variant, Floris, Klum, Landes, Perks, and Psammaquents (Figure 6). The parent material is alluvium, and the soils are moderately well to excessively-drained and deep. Soil pH classes are moderately acid to neutral. No rooting restrictions are noted for the soils of this ecological site (Table 5).
Some soil map units in this ecological site may meet the definition of hydric soils and are listed as meeting criteria 4 of the hydric soils list (77 FR 12234).
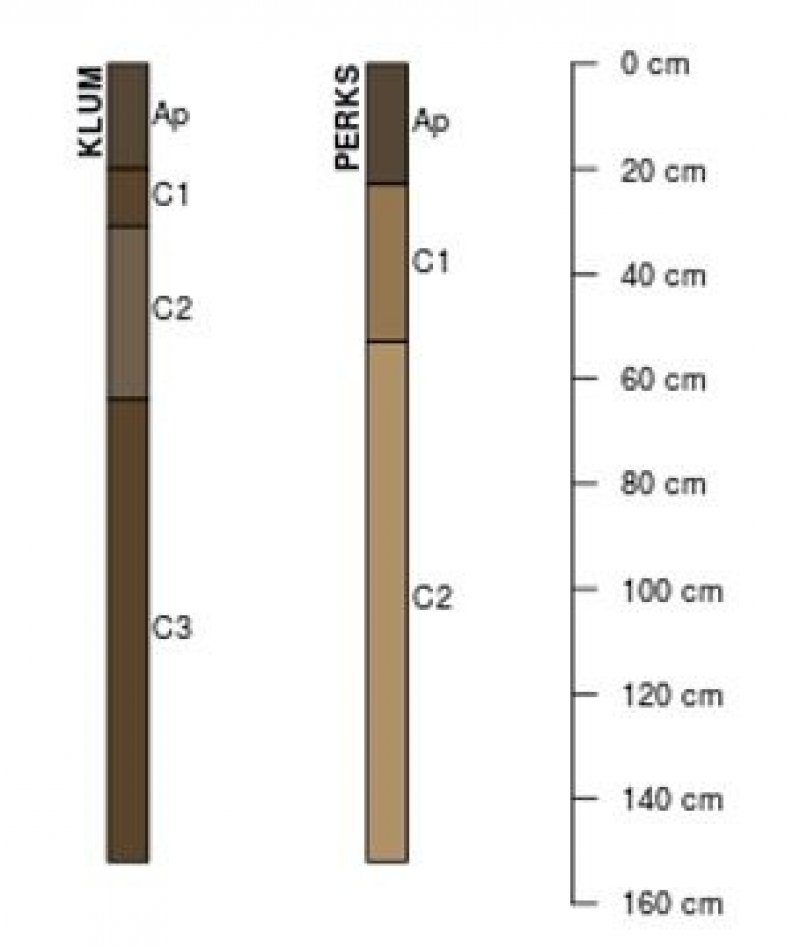
Figure 11. Figure 6. Profile sketches of soil series associated with Sandy Floodplain Forest.
Table 4. Representative soil features
Parent material |
(1)
Alluvium
|
---|---|
Surface texture |
(1) Sandy loam (2) Fine sandy loam (3) Very fine sandy loam (4) Loam |
Family particle size |
(1) Coarse-loamy |
Drainage class | Moderately well drained to excessively drained |
Permeability class | Moderate |
Soil depth | 203 cm |
Surface fragment cover <=3" | 0% |
Surface fragment cover >3" | 0% |
Available water capacity (Depth not specified) |
5.08 – 17.78 cm |
Calcium carbonate equivalent (Depth not specified) |
0 – 20% |
Soil reaction (1:1 water) (Depth not specified) |
5.6 – 7.3 |
Subsurface fragment volume <=3" (Depth not specified) |
0 – 5% |
Subsurface fragment volume >3" (Depth not specified) |
0 – 2% |
Ecological dynamics
The information in this Ecological Site Description, including the state-and-transition model (STM), was developed based on historical data, current field data, professional experience, and a review of the scientific literature. As a result, all possible scenarios or plant species may not be included. Key indicator plant species, disturbances, and ecological processes are described to inform land management decisions.
The MLRA lies within the transition zone between the eastern deciduous forests and the tallgrass prairies. The heterogeneous topography of the area results in variable microclimates and fuel matrices that in turn are able to support prairies, savannas, woodlands, and forests. Sandy Floodplain Forests form an aspect of this vegetative continuum. This ecological site occurs on floodplains on moderately well to excessively-drained soils. Species characteristic of this ecological site consist of hydrophytic woody and herbaceous vegetation.
Flooding is the dominant disturbance factor in Sandy Floodplain Forests. Seasonal flooding occurs every one to two years, and flooding can persist up to 30 days. Floodwaters are fast, and the receding water deposits sediments and debris along the forest floor. Less frequent major and extreme floods result in shifts in the plant community, from early-successional communities with small trees and saplings forming open canopies to late-successional communities with large trees and closed canopies.
Today, many Sandy Floodplain Forests have been reduced as a result of conversion to pasture. A few sites have been cleared and drained for agricultural production. Remnant sites have been degraded due to significant changes to the natural hydrologic regime and water quality in the watershed. The state-and-transition model that follows provides a detailed description of each state, community phase, pathway, and transition. This model is based on available experimental research, field observations, literature reviews, professional consensus, and interpretations.
STATE 1 – REFERENCE STATE
The reference plant community is categorized as a floodplain forest community, dominated by hydrophytic woody and herbaceous vegetation. The three community phases within the reference state are dependent on a regular flood regime. The amount and duration of flooding alters species composition, cover, and extent.
Community Phase 1.1 Silver Maple – American Elm/Riverbank Grape/Whitegrass – Virginia Wildrye – Sites in this reference community phase are a closed canopy forest (80 to 100 percent cover) dominated by silver maple and American elm, with sub-dominants including eastern cottonwood, green ash, slippery elm, boxelder, and common hackberry. Trees are large (21 to 33-inch DBH) and range in height from 30 to over 80 feet tall (LANDFIRE 2009). Climbing vines, especially riverbank grape and bristly greenbriar (Smilax tamnoides L.), can be common. Whitegrass and Virginia wildrye are the dominant herbaceous species, but other species that can be encountered include sweet woodreed (Cinna arundinacea L.), Canadian clearweed (Pilea pumila (L.) A. Gray), Canadian woodnettle (Laportea canadensis (L.) Weddell), and fowl mannagrass (Glyceria striata (Lam.) Hitchc.). Average flood events will likely maintain this phase, but a major or extreme scouring event that removes some to all of the overstory will shift the community to phase 1.3 or 1.2.
Pathway 1.1A – Extreme flood event that removes the overstory.
Pathway 1.1B – Major flood event that reduces the overstory.
Community Phase 1.2 Black Willow/Virginia Wildrye – Devil’s Beggartick – This reference community phase represents a plant community in recovery from an extreme flood event that reduces the overstory canopy. Black willow becomes an important, early-successional woody species to establish on the recently cleared sites (Tesky 1992). Silver maple and American elm saplings can be present. The ground layer is generally sparse with mostly disturbance-tolerant species present, including Virginia wildrye and devil’s beggartick (Bidens frondosa L.). This community phase occurs from the time of disturbance to approximately 20 years (LANDFIRE 2009). Average flood events will slowly shift this community to phase 1.3.
Pathway 1.2A – Natural succession as a result of sediment accumulation from regular flooding.
Community Phase 1.3 Silver Maple/Black Willow/Virginia Wildrye – This reference community phase represents a mid-successional plant community phase. The tree canopy matures out of the sapling phase, with tree size class increasing to medium (9 to 21-inch DBH), cover increasing up to 80 percent, and heights reaching up to 30 feet. Black willow can still be present in the shrub layer, and the herbaceous layer remains sparse. Average flood events will slowly shift this community to phase 1.1 after approximately 60 years (LANDFIRE 2009)
Pathway 1.3A – Natural succession as a result of sediment accumulation from continuous flooding.
Pathway 1.3B – Major flood event that reduces the overstory.
Transition 1A – Altered hydrology throughout the watershed transitions the site to the hydrologically-altered state (2).
Transition 1B – Woody species removal and cultural treatments to enhance forage quality and yield transition the site to the forage state (3).
Transition 1C – Woody species removal, tillage, seeding of agricultural crops, and non-selective herbicide transition the site to the cropland state (4).
STATE 2 – HYDROLOGICALLY-ALTERED STATE
Agricultural tile drainage, stream channelization, and levee construction in hydrologically-connected waters have drastically changed the natural hydrologic regime of Sandy Floodplain Forests. In addition, increased amounts of precipitation and intensity have amplified flooding events (Pryor et al. 2014). This has resulted in a type conversion from the species-rich forest to a ruderal floodplain forest state. In addition, exotic species have encroached and continuously spread, reducing native diversity and ecosystem stability (Eggers and Reed 2015).
Community Phase 2.1 Silver Maple – Boxelder/Common Hackberry – Black Walnut/Creeping Jenny – This community phase represents a shift in plant community composition as a result of an altered hydrologic regime. Silver maple is still dominant, but the ruderal boxelder can begin to co-dominate. A reduced flooding frequency from stream channelization and water control structures allows other species to become prominent in the canopy composition including common hackberry, black walnut (Juglans nigra L.), honeylocust (Gleditsia triacanthos L.) and bitternut hickory (Carya cordiformis (Wangenh.) K. Koch). The bare soil conditions that result from flooding allow non-native species – such as Creeping jenny (Lysimachia nummularia L.) – to rapidly colonize and expand in the understory.
Pathway 2.1A – Continuing hydrologic alterations within the watershed.
Community Phase 2.2 Common Hackberry – Black Walnut/Multiflora Rose – Roughleaf Dogwood/Creeping Jenny – This community phase represents persisting changes to the natural hydrology of the watershed. The overstory canopy continues to shift, oftentimes becoming dominated by common hackberry and black walnut. Multiflora rose (Rosa multiflora L.) and roughleaf dogwood (Cornus drummondii C.A. Mey) are common in the shrub layer.
Transition 2A – Woody species removal and cultural treatments to enhance forage quality and yield transition the site to the forage state (3).
Transition 2B – Woody species removal, tillage, seeding of agricultural crops, and non-selective herbicide transition the site to the cropland state (4).
Restoration 2A – Site preparation, tree planting, timber stand improvement, non-native species control, and water control structures installed to improve and regulate hydrology transition this site to the reconstructed forest state (5).
STATE 3 – FORAGE STATE
The forage state arises when the site is converted to a farming system that emphasizes domestic livestock production, known as grassland agriculture. Fire suppression, periodic cultural treatments (e.g., clipping, drainage, soil amendment applications, planting new species and/or cultivars, mechanical harvesting) and grazing by domesticated livestock transition and maintain this state (USDA-NRCS 2003). Early settlers seeded non-native species, as smooth brome (Bromus inermis Leyss.) and Kentucky bluegrass (Poa pratensis L.), to help extend the grazing season (Smith 1998). Over time, as lands were continuously harvested or grazed by herds of cattle, these species were able to spread and expand across the landscape, reducing the native species diversity and ecological function.
Community Phase 3.1 Hayfield – Sites in this community phase consist of forage plants that are planted and mechanically harvested. Mechanical harvesting removes a significant portion of the aboveground biomass and nutrients that feed the soil microorganisms (Franzluebbers et al. 2000; USDA-NRCS 2003). As a result, soil biology is reduced leading to decreases in nutrient uptake by plants, soil organic matter, and soil aggregation. Frequent biomass removal can also reduce the site’s carbon sequestration capacity (Skinner 2008).
Pathway 3.1A – Mechanical harvesting is replaced with domestic livestock utilizing continuous grazing.
Pathway 3.1B – Mechanical harvesting is replaced with domestic livestock utilizing rotational grazing.
Community Phase 3.2 Continuous Pastured Grazing System – This community phase is characterized by continuous grazing where domestic livestock graze a pasture for the entire season. Depending on stocking density, this can result in lower forage quality and productivity, weed invasions, and uneven pasture use. Continuous grazing can also increase the amount of bare ground and erosion and reduce soil organic matter, cation exchange capacity, water-holding capacity, and nutrient availability and retention (Bharati et al. 2002; Leake et al. 2004; Teague et al. 2011). Smooth brome, Kentucky bluegrass, and white clover (Trifolium repens L.) are common pasture species used in this phase. Their tolerance to continuous grazing has allowed these species to dominate, sometimes completely excluding the native vegetation.
Pathway 3.2A – Domestic livestock are removed and mechanical harvesting is implemented.
Pathway 3.2B – Rotational grazing replaces continuous grazing.
Community Phase 3.3 Rest-Rotation Pastured Grazing System – This community phase is characterized by rotational grazing where the pasture has been subdivided into several smaller paddocks. Through the development of a grazing plan, livestock utilize one or a few paddocks, while the remaining area is rested allowing plants to restore vigor and energy reserves, deepen root systems, develop seeds, as well as allow seedling establishment (Undersander et al. 2002; USDA-NRCS 2003). Rest-rotation pastured grazing systems include deferred rotation, rest rotation, high intensity – low frequency, and short duration methods. Vegetation is generally more diverse and can include orchardgrass (Dactylis glomerata L.), timothy (Phleum pretense L.), red clover (Trifolium pratense L.), and alfalfa (Medicago sativa L.). The addition of native prairie species can further bolster plant diversity and, in turn, soil function. This community phase promotes numerous ecosystem benefits including increasing biodiversity, preventing soil erosion, maintaining and enhancing soil quality, sequestering atmospheric carbon, and improving water yield and quality (USDA-NRCS 2003).
Pathway 3.3A – Continuous grazing replaces rotational grazing.
Pathway 3.3B – Domestic livestock are removed and mechanical harvesting is implemented.
Transition 3A – Land is abandoned and left fallow; natural succession by opportunistic species transition this site the hydrologically-altered state (2).
Transition 3B – Tillage, seeding of agricultural crops, and non-selective herbicide transition the site to the cropland state (4).
Restoration 3A – Site preparation, tree planting, timber stand improvement, non-native species control, and water control structures installed to improve and regulate hydrology transition this site to the reconstructed forest state (5).
STATE 4 – CROPLAND STATE
The Midwest is well-known for its highly-productive agricultural soils, and as a result, much of the MLRA has been converted to cropland, including portions of this ecological site. The continuous use of tillage, row-crop planting, and chemicals (i.e., herbicides, fertilizers, etc.) has effectively eliminated the reference community and many of its natural ecological functions in favor of crop production. Corn (Zea mays L.) and soybeans (Glycine max (L.) Merr.) are the dominant crops for the site. These areas are likely to remain in crop production for the foreseeable future.
Community Phase 4.1 Conventional Tillage Field – Sites in this community phase typically consist of monoculture row-cropping maintained by conventional tillage practices. They are cropped in either continuous corn or corn-soybean rotations. The frequent use of deep tillage, low crop diversity, and bare soil conditions during the non-growing season negatively impacts soil health. Under these practices, soil aggregation is reduced or destroyed, soil organic matter is reduced, erosion and runoff are increased, and infiltration is decreased, which can ultimately lead to undesirable changes in the hydrology of the watershed (Tomer et al. 2005).
Pathway 4.1A – Tillage operations are greatly reduced, crop rotation occurs on a regular schedule, and crop residue is allowed to remain on the soil surface.
Pathway 4.1B – Tillage operations are greatly reduced or eliminated, crop rotation is either reduced or eliminated, and crop residue is allowed to remain on the soil surface, and cover crops are implemented to prevent soil erosion.
Community Phase 4.2 Conservation Tillage Field – This community phase is characterized by rotational crop production that utilizes various conservation tillage methods to promote soil health and reduce erosion. Conservation tillage methods include strip-till, ridge-till, vertical-till, or no-till planting systems. Strip-till keeps seedbed preparation to narrow bands less than one-third the width of the row where crop residue and soil consolidation are left undisturbed in-between seedbed areas. Strip-till planting may be completed in the fall and nutrient application either occurs simultaneously or at the time of planting. Ridge-till uses specialized equipment to create ridges in the seedbed and vegetative residue is left on the surface in between the ridges. Weeds are controlled with herbicides and/or cultivation, seedbed ridges are rebuilt during cultivation, and soils are left undisturbed from harvest to planting. Vertical-till systems employ machinery that lightly tills the soil and cuts up crop residue, mixing some of the residue into the top few inches of the soil while leaving a large portion on the surface. No-till management is the most conservative, disturbing soils only at the time of planting and fertilizer application. Compared to conventional tillage systems, conservation tillage methods can reduce soil erosion, increase organic matter and water availability, improve water quality, and reduce soil compaction.
Pathway 4.2A – Intensive tillage is utilized and monoculture row-cropping is established.
Pathway 4.2B – Cover crops are implemented to prevent soil erosion.
Community Phase 4.3 Conservation Tillage with Cover Crop Field – This condition applies conservation tillage methods as described above as well as adds cover crop practices. Cover crops typically include nitrogen-fixing species (e.g., legumes), small grains (e.g., rye, wheat, oats), or forage covers (e.g., turnips, radishes, rapeseed). The addition of cover crops not only adds plant diversity but also promotes soil health by reducing soil erosion, limiting nitrogen leaching, suppressing weeds, increasing soil organic matter, and improving the overall soil. In the case of small grain cover crops, surface cover and water infiltration are increased, while forage covers can be used to graze livestock or support local wildlife. Of the three community phases for this state, this phase promotes the greatest soil sustainability and improves ecological functioning within a cropland system.
Pathway 4.3A – Cover crop practices are abandoned.
Pathway 4.3B – Intensive tillage is utilized, cover crops practices are abandoned, monoculture row-cropping is established, and crop rotation is reduced or eliminated.
Transition 4A – Land abandonment transitions the site to the hydrologically-altered state (2).
Transition 4B – Cultural treatments to enhance forage quality and yield transition the site to the forage state (3).
Restoration 4A – Site preparation, tree planting, timber stand improvement, non-native species control, and water control structures installed to improve and regulate hydrology transition this site to the reconstructed forest state (5).
STATE 5 – RECONSTRUCTED FLOODPLAIN FOREST STATE
The combination of natural and anthropogenic disturbances occurring today has resulted in numerous ecosystem health issues, and restoration back to the historic reference state may not be possible. Many natural forest communities are being stressed by non-native diseases and pests, habitat fragmentation, permanent changes in hydrologic regimes, and overabundant deer populations on top of naturally-occurring disturbances (severe weather and native pests) (Flickinger 2010). However, these habitats provide multiple ecosystem services including carbon sequestration; clean air and water; soil conservation; biodiversity support; wildlife habitat; as well as a variety of cultural activities (e.g., hiking, hunting) (Millennium Ecosystem Assessment 2005; Flickinger 2010). Therefore, conservation of floodplain forests should still be pursued. Habitat reconstructions are an important tool for repairing natural ecological functioning and providing habitat protection for numerous species of Sandy Floodplain Forests. Therefore, ecological restoration should aim to aid the recovery of degraded, damaged, or destroyed ecosystems. A successful restoration will have the ability to structurally and functionally sustain itself, demonstrate resilience to the ranges of stress and disturbance, and create and maintain positive biotic and abiotic interactions (SER 2002). The reconstructed forest state is the result of a long-term commitment involving a multi-step, adaptive management process.
Community Phase 5.1 Early Successional Reconstructed Forest – This community phase represents the early community assembly from forest reconstruction. It is highly dependent on the current condition of the site based on past and current land management actions, invasive species, and proximity to land populated with non-native pests and diseases. Therefore, no two sites will have the same early successional composition. Technical forestry assistance should be sought to develop suitable conservation management plans.
Pathway 5.1A – Application of stand improvement practices in line with a developed management plan.
Community Phase 5.2 Late Successional Reconstructed Forest – Appropriately timed management practices (e.g. forest stand improvement, continuing integrated pest management) applied to the early successional community phase can help increase the stand maturity, pushing the site into a late successional community phase over time. A late successional reconstructed forest will have an uneven-aged, closed canopy and a well-developed understory.
Pathway 5.2A – Reconstruction experiences a setback from extreme weather event or improper timing of management actions.
Transition 5A – Removal of water control structures and unmanaged invasive species populations transition this site to the hydrologically-altered state (2).
Transition 5B – Tree removal and cultural treatments to enhance forage quality and yield transition the site to the forage state (3).
Transition 5C – Tree removal, tillage, seeding of agricultural crops, and non-selective herbicide transition this site to the cropland state (4).
State and transition model
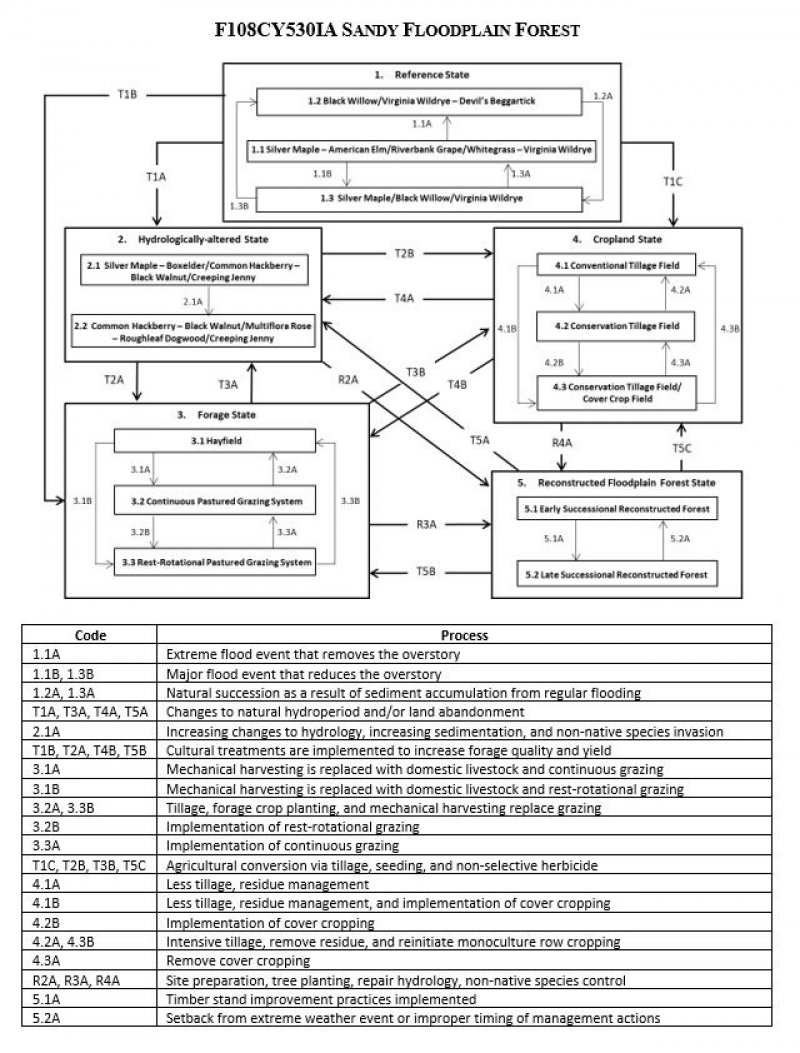
More interactive model formats are also available.
View Interactive Models
More interactive model formats are also available.
View Interactive Models
Click on state and transition labels to scroll to the respective text
Ecosystem states
States 2 and 5 (additional transitions)
State 3 submodel, plant communities
State 4 submodel, plant communities
State 5 submodel, plant communities
State 1
Reference State
The reference plant community is categorized as a floodplain forest community, dominated by hydrophytic woody and herbaceous vegetation. The three community phases within the reference state are dependent on a regular flood regime. The amount and duration of flooding alters species composition, cover, and extent.
Community 1.1
Silver Maple – American Elm/Riverbank Grape/Whitegrass – Virginia Wildrye
Sites in this reference community phase are a closed canopy forest (80 to 100 percent cover) dominated by silver maple and American elm, with sub-dominants including eastern cottonwood, green ash, slippery elm, boxelder, and common hackberry. Trees are large (21 to 33-inch DBH) and range in height from 30 to over 80 feet tall (LANDFIRE 2009). Climbing vines, especially riverbank grape and bristly greenbriar (Smilax tamnoides L.), can be common. Whitegrass and Virginia wildrye are the dominant herbaceous species, but other species that can be encountered include sweet woodreed (Cinna arundinacea L.), Canadian clearweed (Pilea pumila (L.) A. Gray), Canadian woodnettle (Laportea canadensis (L.) Weddell), and fowl mannagrass (Glyceria striata (Lam.) Hitchc.). Average flood events will likely maintain this phase, but a major or extreme scouring event that removes some to all of the overstory will shift the community to phase 1.3 or 1.2.
Community 1.2
Black Willow/Virginia Wildrye – Devil’s Beggartick
This reference community phase represents a plant community in recovery from an extreme flood event that reduces the overstory canopy. Black willow becomes an important, early-successional woody species to establish on the recently cleared sites (Tesky 1992). Silver maple and American elm saplings can be present. The ground layer is generally sparse with mostly disturbance-tolerant species present, including Virginia wildrye and devil’s beggartick (Bidens frondosa L.). This community phase occurs from the time of disturbance to approximately 20 years (LANDFIRE 2009). Average flood events will slowly shift this community to phase 1.3.
Community 1.3
Silver Maple/Black Willow/Virginia Wildrye
This reference community phase represents a mid-successional plant community phase. The tree canopy matures out of the sapling phase, with tree size class increasing to medium (9 to 21-inch DBH), cover increasing up to 80 percent, and heights reaching up to 30 feet. Black willow can still be present in the shrub layer, and the herbaceous layer remains sparse. Average flood events will slowly shift this community to phase 1.1 after approximately 60 years (LANDFIRE 2009)
Pathway 1.1A
Community 1.1 to 1.2
Extreme flood event that removes the overstory.
Pathway 1.1B
Community 1.1 to 1.3
Major flood event that reduces the overstory.
Pathway 1.2A
Community 1.2 to 1.3
Natural succession as a result of sediment accumulation from regular flooding.
Pathway 1.3A
Community 1.3 to 1.1
Natural succession as a result of sediment accumulation from continuous flooding.
Pathway 1.3B
Community 1.3 to 1.2
Major flood event that reduces the overstory.
State 2
Hydrologically-altered State
Agricultural tile drainage, stream channelization, and levee construction in hydrologically-connected waters have drastically changed the natural hydrologic regime of Sandy Floodplain Forests. In addition, increased amounts of precipitation and intensity have amplified flooding events (Pryor et al. 2014). This has resulted in a type conversion from the species-rich forest to a ruderal floodplain forest state. In addition, exotic species have encroached and continuously spread, reducing native diversity and ecosystem stability (Eggers and Reed 2015).
Community 2.1
Silver Maple – Boxelder/Common Hackberry – Black Walnut/Creeping Jenny
This community phase represents a shift in plant community composition as a result of an altered hydrologic regime. Silver maple is still dominant, but the ruderal boxelder can begin to co-dominate. A reduced flooding frequency from stream channelization and water control structures allows other species to become prominent in the canopy composition including common hackberry, black walnut (Juglans nigra L.), honeylocust (Gleditsia triacanthos L.) and bitternut hickory (Carya cordiformis (Wangenh.) K. Koch). The bare soil conditions that result from flooding allow non-native species – such as Creeping jenny (Lysimachia nummularia L.) – to rapidly colonize and expand in the understory.
Community 2.2
Common Hackberry – Black Walnut/Multiflora Rose – Roughleaf Dogwood/Creeping Jenny
This community phase represents persisting changes to the natural hydrology of the watershed. The overstory canopy continues to shift, oftentimes becoming dominated by common hackberry and black walnut. Multiflora rose (Rosa multiflora L.) and roughleaf dogwood (Cornus drummondii C.A. Mey) are common in the shrub layer.
Pathway 2.1A
Community 2.1 to 2.2
Continuing hydrologic alterations within the watershed.
State 3
Forage State
The forage state occurs when the site is converted to a farming operation that emphasizes domestic livestock production known as grassland agriculture. Fire suppression, periodic cultural treatments (e.g., clipping, drainage, soil amendment applications, planting new species and/or cultivars, mechanical harvesting) and grazing by domesticated livestock transition and maintain this state (USDA-NRCS 2003). Early settlers seeded non-native species, such as smooth brome (Bromus inermis Leyss.) and Kentucky bluegrass (Poa pratensis L.), to help extend the grazing season (Smith 1998). Over time, as lands were continuously harvested or grazed by herds of cattle, the non-native species were able to spread and expand across the landscape, reducing the native species diversity and ecological function.
Community 3.1
Hayfield
Sites in this community phase consist of forage plants that are planted and mechanically harvested. Mechanical harvesting removes much of the aboveground biomass and nutrients that feed the soil microorganisms (Franzluebbers et al. 2000; USDA-NRCS 2003). As a result, soil biology is reduced leading to decreases in nutrient uptake by plants, soil organic matter, and soil aggregation. Frequent biomass removal can also reduce the site’s carbon sequestration capacity (Skinner 2008).
Community 3.2
Continuous Pastured Grazing
This community phase is characterized by continuous grazing where domestic livestock graze a pasture for the entire season. Depending on stocking density, this can result in lower forage quality and productivity, weed invasions, and uneven pasture use. Continuous grazing can also increase the amount of bare ground and erosion and reduce soil organic matter, cation exchange capacity, water-holding capacity, and nutrient availability and retention (Bharati et al. 2002; Leake et al. 2004; Teague et al. 2011). Smooth brome, Kentucky bluegrass, and white clover (Trifolium repens L.) are common pasture species used in this phase. Their tolerance to continuous grazing has allowed these species to dominate, sometimes completely excluding the native vegetation.
Community 3.3
Periodic-rest Pastured Grazing
This community phase is characterized by periodic-rest grazing where the pasture has been subdivided into several smaller paddocks. Subdividing the pasture in this way allows livestock to utilize one or a few paddocks, while the remaining area is rested allowing plants to restore vigor and energy reserves, deepen root systems, develop seeds, as well as allow seedling establishment (Undersander et al. 2002; USDA-NRCS 2003). Periodic-rest pastured grazing includes deferred periods, rest periods, and periods of high intensity – low frequency, and short duration methods. Vegetation is generally more diverse and can include orchardgrass (Dactylis glomerata L.), timothy (Phleum pretense L.), red clover (Trifolium pratense L.), and alfalfa (Medicago sativa L.). The addition of native prairie species can further bolster plant diversity and, in turn, soil function. This community phase promotes numerous ecosystem benefits including increasing biodiversity, preventing soil erosion, maintaining and enhancing soil quality, sequestering atmospheric carbon, and improving water yield and quality (USDA-NRCS 2003).
Pathway 3.1A
Community 3.1 to 3.2
Mechanical harvesting is replaced with domestic livestock utilizing continuous grazing.
Pathway 3.1B
Community 3.1 to 3.3
Mechanical harvesting is replaced with domestic livestock utilizing periodic-rest grazing.
Pathway 3.2A
Community 3.2 to 3.1
Domestic livestock are removed, and mechanical harvesting is implemented.
Pathway 3.2B
Community 3.2 to 3.3
Periodic-rest grazing replaces continuous grazing.
Pathway 3.3B
Community 3.3 to 3.1
Domestic livestock are removed, and mechanical harvesting is implemented.
Pathway 3.3A
Community 3.3 to 3.2
Continuous grazing replaces periodic-rest grazing.
State 4
Cropland State
The low topographic relief across the MLRA has resulted in nearly the entire area being converted to agriculture (Eilers and Roosa 1994). The continuous use of tillage, row-crop planting, and chemicals (i.e., herbicides, fertilizers, etc.) has effectively eliminated the reference community and many of its natural ecological functions in favor of crop production. Corn and soybeans are the dominant crops for the site, and oats (Avena L.) and alfalfa (Medicago sativa L.) may be rotated periodically. These areas are likely to remain in crop production for the foreseeable future.
Community 4.1
Conventional Tillage Field
Sites in this community phase typically consist of monoculture row-cropping maintained by conventional tillage practices. They are cropped in either continuous corn or alternating periods of corn and soybean crops. The frequent use of deep tillage, low crop diversity, and bare soil conditions during the non-growing season negatively impacts soil health. Under these practices, soil aggregation is reduced or destroyed, soil organic matter is reduced, erosion and runoff are increased, and infiltration is decreased, which can ultimately lead to undesirable changes in the hydrology of the watershed (Tomer et al. 2005).
Community 4.2
Conservation Tillage Field
This community phase is characterized by periodically alternating crops and utilizing various conservation tillage methods to promote soil health and reduce erosion. Conservation tillage methods include strip-till, ridge-till, vertical-till, or no-till planting operations. Strip-till keeps seedbed preparation to narrow bands less than one-third the width of the row where crop residue and soil consolidation are left undisturbed in-between seedbed areas. Strip-till planting may be completed in the fall and nutrient application either occurs simultaneously or at the time of planting. Ridge-till uses specialized equipment to create ridges in the seedbed and vegetative residue is left on the surface in between the ridges. Weeds are controlled with herbicides and/or cultivation, seedbed ridges are rebuilt during cultivation, and soils are left undisturbed from harvest to planting. Vertical-till operations employ machinery that lightly tills the soil and cuts up crop residue, mixing some of the residue into the top few inches of the soil while leaving a large portion on the surface. No-till management is the most conservative, disturbing soils only at the time of planting and fertilizer application. Compared to conventional tillage operations, conservation tillage methods can improve soil ecosystem function by reducing soil erosion, increasing organic matter and water availability, improving water quality, and reducing soil compaction.
Community 4.3
Conservation Tillage with Cover Crop Field
This community phase applies conservation tillage methods as described above as well as adds cover crop practices. Cover crops typically include nitrogen-fixing species (e.g., legumes), small grains (e.g., rye, wheat, oats), or forage covers (e.g., turnips, radishes, rapeseed). The addition of cover crops not only adds plant diversity but also promotes soil health by reducing soil erosion, limiting nitrogen leaching, suppressing weeds, increasing soil organic matter, and improving the overall soil ecosystem. In the case of small grain cover crops, surface cover and water infiltration are increased, while forage covers can be used to graze livestock or support local wildlife. Of the three community phases for this state, this phase promotes the greatest soil sustainability and improves ecological functioning within a row crop operation.
Pathway 4.1A
Community 4.1 to 4.2
Tillage operations are greatly reduced, alternating crops occurs on a regular interval, and crop residue remains on the soil surface.
Pathway 4.1B
Community 4.1 to 4.3
Tillage operations are greatly reduced or eliminated, alternating crops occurs on a regular interval, crop residue remains on the soil surface, and cover crops are planted following crop harvest.
Pathway 4.2A
Community 4.2 to 4.1
Intensive tillage is utilized, and monoculture row-cropping is established.
Pathway 4.2B
Community 4.2 to 4.3
Cover crops are implemented to minimize soil erosion.
Pathway 4.3B
Community 4.3 to 4.1
Intensive tillage is utilized, cover crops practices are abandoned, monoculture row-cropping is established on a more-or-less continuous basis.
Pathway 4.3A
Community 4.3 to 4.2
Cover crop practices are abandoned.
State 5
Reconstructed Floodplain Forest State
The combination of natural and anthropogenic disturbances occurring today has resulted in numerous ecosystem health issues, and restoration back to the historic reference state may not be possible. Many natural forest communities are being stressed by non-native diseases and pests, habitat fragmentation, permanent changes in hydrologic regimes, and overabundant deer populations on top of naturally-occurring disturbances (severe weather and native pests) (Flickinger 2010). However, these habitats provide multiple ecosystem services including carbon sequestration; clean air and water; soil conservation; biodiversity support; wildlife habitat; as well as a variety of cultural activities (e.g., hiking, hunting) (Millennium Ecosystem Assessment 2005; Flickinger 2010). Therefore, conservation of floodplain forests should still be pursued. Habitat reconstructions are an important tool for repairing natural ecological functioning and providing habitat protection for numerous species of Sandy Floodplain Forests. Therefore, ecological restoration should aim to aid the recovery of degraded, damaged, or destroyed ecosystems. A successful restoration will have the ability to structurally and functionally sustain itself, demonstrate resilience to the ranges of stress and disturbance, and create and maintain positive biotic and abiotic interactions (SER 2002). The reconstructed forest state is the result of a long-term commitment involving a multi-step, adaptive management process.
Community 5.1
Early Successional Reconstructed Forest
This community phase represents the early community assembly from forest reconstruction. It is highly dependent on the current condition of the site based on past and current land management actions, invasive species, and proximity to land populated with non-native pests and diseases. Therefore, no two sites will have the same early successional composition. Technical forestry assistance should be sought to develop suitable conservation management plans.
Community 5.2
Late Successional Reconstructed Forest
Appropriately timed management practices (e.g. forest stand improvement, continuing integrated pest management) applied to the early successional community phase can help increase the stand maturity, pushing the site into a late successional community phase over time. A late successional reconstructed forest will have an uneven-aged, closed canopy and a well-developed understory.
Pathway 5.1A
Community 5.1 to 5.2
Application of stand improvement practices in line with a developed management plan.
Pathway 5.2A
Community 5.2 to 5.1
Reconstruction experiences a setback from extreme weather event or improper timing of management actions.
Transition T1A
State 1 to 2
Altered hydrology throughout the watershed transitions the site to the hydrologically-altered state (2).
Transition T1B
State 1 to 3
Woody species removal and cultural treatments to enhance forage quality and yield transition the site to the forage state (3).
Transition T1C
State 1 to 4
Woody species removal, tillage, seeding of agricultural crops, and non-selective herbicide transition the site to the cropland state (4).
Transition T2A
State 2 to 3
Woody species removal and cultural treatments to enhance forage quality and yield transition the site to the forage state (3).
Transition T2B
State 2 to 4
Woody species removal, tillage, seeding of agricultural crops, and non-selective herbicide transition the site to the cropland state (4).
Restoration pathway R2A
State 2 to 5
Site preparation, tree planting, timber stand improvement, non-native species control, and water control structures installed to improve and regulate hydrology transition this site to the reconstructed forest state (5).
Transition T3A
State 3 to 2
Land abandonment transitions the site to the hydrologically-altered state (2).
Transition T3B
State 3 to 4
Tillage, seeding of agricultural crops, and non-selective herbicide transition this site to the cropland state (4).
Restoration pathway R3A
State 3 to 5
Site preparation, tree planting, timber stand improvement, non-native species control, and water control structures installed to improve and regulate hydrology transition this site to the reconstructed forest state (5).
Transition T4A
State 4 to 2
Land abandonment transitions the site to the hydrologically-altered state (2).
Transition T4B
State 4 to 3
Cultural treatments to enhance forage quality and yield transitions the site to the forage state (3).
Restoration pathway R4A
State 4 to 5
Site preparation, tree planting, timber stand improvement, non-native species control, and water control structures installed to improve and regulate hydrology transition this site to the reconstructed forest state (5).
Transition T5A
State 5 to 2
Removal of water control structures and unmanaged invasive species populations transition this site to the hydrologically-altered state (2).
Transition T5B
State 5 to 3
Tree removal and cultural treatments to enhance forage quality and yield transition the site to the forage state (3).
Transition T5C
State 5 to 4
Tree removal, tillage, seeding of agricultural crops, and non-selective herbicide transition this site to the cropland state (4).
Additional community tables
Interpretations
Supporting information
Inventory data references
Tier 3 Sampling Plot used to develop the alternative state, community phase 2.2:
State County Ownership Legal Description Easting Northing
Iowa Clinton Wapsie Environmental Center – Scott & Clinton County Conservation Boards T81N R1E S36 683904 4626864
Other references
Baker, R.G., C.A. Chumbley, P.M. Witinok, and H.K. Kim. 1990. Holocene vegetational changes in eastern Iowa. Journal of the Iowa Academy of Science 97: 167-177.
Baker, R.G., L.J. Maher, C.A. Chumbley, and K.L. Van Zant. 1992. Patterns of Holocene environmental changes in the midwestern United States. Quaternary Research 37: 379-389.
Bharati, L., K.-H. Lee, T.M. Isenhart, and R.C. Schultz. 2002. Soil-water infiltration under crops, pasture, and established riparian buffer in Midwestern USA. Agroforestry Systems 56: 249-257.
Changes in Hydric Soils Database Selection Criteria. 77 Federal Register 12234 (29 February 2012), pp. 12234-12235.
Cleland, D.T., J.A. Freeouf, J.E. Keys, G.J. Nowacki, C. Carpenter, and W.H. McNab. 2007. Ecological Subregions: Sections and Subsections of the Coterminous United States. USDA Forest Service, General Technical Report WO-76. Washington, DC. 92 pps.
Eggers, S.D. and D.M. Reed. 2015. Wetland Plants and Plant Communities of Minnesota and Wisconsin, Version 3.2. U.S. Army Corps of Engineers, Regulatory Branch, St. Paul District. St. Paul, MN. 478 pps.
Federal Geographic Data Committee. 2013. Classification of Wetlands and Deepwater Habitats of the United States. FGDC-STD-004-2013. Second Edition. Wetlands Subcommittee, Federal geographic Data Committee and U.S. Fish and Wildlife Service, Washington, D.C. 90 pps.
Flickinger, A. 2010. Iowa Forests Today: An Assessment of the Issues and Strategies for Conserving and Managing Iowa’s Forests. Iowa Department of Natural Resources. 329 pps.
Franzluebbers, A.J., J.A. Stuedemann, H.H. Schomberg, and S.R. Wilkinson. 2000. Soil organic C and N pools under long-term pasture management in the Southern Piedmont USA. Soil Biology and Biochemistry 32:469-478.
Iowa Natural Areas Inventory [INAI]. 1984. An Inventory of Significant Natural Areas in Iowa: Two Year Progress Report of the Iowa Natural Areas Inventory. Iowa Natural Areas Inventory, Iowa Department of Natural Resources, Des Moines, IA.
LANDFIRE. 2009. Biophysical Setting 4214710 Central Interior and Appalachian Floodplain Systems. In: LANDFIRE National Vegetation Dynamics Models. USDA Forest Service and US Department of Interior. Washington, DC.
Leake, J., D. Johnson, D. Donnelly, G. Muckle, L. Boddy, and D. Read. 2004. Networks of power and influence: the role of mycorrhizal mycelium in controlling plant communities and agroecosystem functioning. Canadian Journal of Botany 82: 1016-1045.
Millennium Ecosystem Assessment. 2005. Ecosystems and Human Well-Being: Current States and Trends. World Resources Institute. Island Press, Washington, D.C. 948 pages.
National Climate Data Center [NCDC]. 2006. Climate of Iowa. Central Region Headquarters, Climate Services Branch, National Climatic Data Center, Asheville, NC.
NatureServe. 2015. NatureServe Explorer: An online encyclopedia of life [web application]. Version 7.1 NatureServe, Arlington, VA. Available at http://explorer.natureserve.org. (Accessed 13 February 2017).
Peel, M.C., B.L. Finlayson, and T.A. McMahon. 2007. Updated world map of the Köppen-Geiger climate classification. Hydrology and Earth System Sciences 11: 1633-1644.
Prior, J.C. 1991. Landforms of Iowa. University of Iowa Press for the Iowa Department of Natural Resources, Iowa City, IA. 153 pps.
Pryor, S.C., D. Scavia, C. Downer, M. Gaden, L. Iverson, R. Nordstrom, J. Patz, and G.P. Robertson. 2014. Chapter 18: Midwest. In: J.M. Melillo, T.C. Richmond, and G.W. Yohe, eds. Climate Change Impacts in the United States: The Third National Climate Assessment. U.S. Global Change Research Program, 418-440. Doi:10.7930/J0J1012N.
Skinner, R.H. 2008. High biomass removal limits carbon sequestration potential of mature temperate pastures. Journal for Environmental Quality 37: 1319-1326.
Smith, R.D., A. Ammann, C. Bartoldus, and M.M. Brinson. 1995. An Approach for Assessing Wetland Functions Using Hydrogeomorphic Classification, Reference Wetlands, and Functional Indices. U.S. Army Corps of Engineers, Waterways Experiment Station, Wetlands Research Program Technical Report WRP-DE-9. 78 pps.
Society for Ecological Restoration [SER] Science & Policy Working Group. 2002. The SER Primer on Ecological Restoration. Available at: http://www.ser.org/. (Accessed 28 February 2017).
Teague, W.R., S.L. Dowhower, S.A. Baker, N. Haile, P.B. DeLaune, and D.M. Conover. 2011. Grazing management impacts on vegetation, soil biota and soil chemical, physical and hydrological properties in tall grass prairie. Agriculture, Ecosystems and Environment 141: 310-322.
Tesky, J.L. 1992. Salix nigra. In: Fire Effects Information System [Online]. U.S. Department of Agriculture, Forest Service, Rocky Mountain Research Station, Fire Sciences Laboratory. Available at: https://www.crs-feis.org/feis. (Accessed 6 March 2018).
Tomer, M.D., D.W. Meek, and L.A. Kramer. 2005. Agricultural practices influence flow regimes of headwater streams in western Iowa. Journal of Environmental Quality 34:1547-1558.
Undersander, D., B. Albert, D. Cosgrove, D. Johnson, and P. Peterson. 2002. Pastures for Profit: A Guide to Rotational Grazing (A3529). University of Wisconsin-Extension and University of Minnesota Extension Service. 43 pps.
U.S. Army Corps of Engineers [USACE]. 2010. Regional Supplement to the Corps of Engineers Wetland Delineation Manual: Midwest Region (Version 2.0). U.S. Army Corps of Engineers, Wetlands Regulatory Assistance Program, U.S. Army Engineer Research and Development Center, Vicksburg, MS. 141 pps.
United States Department of Agriculture – Natural Resources Conservation Service (USDA-NRCS). 2003. National Range and Pasture Handbook, Revision 1. Grazing Lands Technology Institute. 214 pps.
United States Department of Agriculture – Natural Resource Conservation Service (USDA-NRCS). 2006. Land Resource Regions and Major Land Resource Areas of the United States, the Caribbean, and the Pacific Basin. U.S. Department of Agriculture Handbook 296. 682 pps.
United States Department of Agriculture – Natural Resources Conservation Service (USDA-NRCS). 2007. Iowa NRCS Plant Community Species Lists. Des Moines, IA. Available at https://www.nrcs.usda.gov/wps/ portal/nrcs/detail/ia/technical/ecoscience/bio/?cid=nrcs142p2_008160. (Accessed 19 January 2018).
United States Department of Agriculture – Natural Resource Conservation Service (USDA-NRCS). 2008. Hydrogeomorphic Wetland Classification: An Overview and Modification to Better Meet the Needs of the Natural Resources Conservation Service. Technical Note No. 190-8-76. Washington, D.C. 8 pps.
U.S. Environmental Protection Agency [EPA]. 2013. Level III and Level IV Ecoregions of the Continental United States. Corvallis, OR, U.S. EPA, National Health and Environmental Effects Research Laboratory, map scale 1: 3,000,000. Available at http://www.epa.gov/eco-research/level-iii-andiv-ecoregions-continental-united-states. (Accessed 1 March 2017).
Contributors
Lisa Kluesner
Ryan Dermody
Approval
Suzanne Mayne-Kinney, 11/04/2024
Acknowledgments
This project could not have been completed without the dedication and commitment from a variety of partners and staff (Table 6). Team members supported the project by serving on the technical team, assisting with the development of state and community phases of the state-and-transition model, providing peer review and technical editing, and conducting quality control and quality assurance reviews.
Organization Name Title Location
Drake University:
Dr. Tom Rosburg Professor of Ecology and Botany Des Moines, IA
Iowa Department of Natural Resources:
Kevin Andersen Private Lands Biologist Fairfield, IA
John Pearson Ecologist Des Moines, IA
LANDFIRE (The Nature Conservancy):
Randy Swaty Ecologist Evanston, IL
Natural Resources Conservation Service:
Rick Bednarek Iowa State Soil Scientist Des Moines, IA
Leland Camp Soil Scientist Waverly, IA
Stacey Clark Regional Ecological Site Specialist St. Paul, MN
Ryan Dermody Soil Survey Leader Waverly, IA
Tonie Endres Senior Regional Soil Scientist Indianapolis, IN
John Hammerly Soil Data Quality Specialist Indianapolis, IN
Lisa Kluesner Ecological Site Specialist Waverly, IA
Sean Kluesner Earth Team Volunteer Waverly, IA
Jeff Matthias State Grassland Specialist Des Moines, IA
Kevin Norwood Soil Survey Regional Director Indianapolis, IN
Doug Oelmann Soil Scientist Des Moines, IA
James Phillips GIS Specialist Des Moines, IA
Jason Steele Area Resource Soil Scientist Fairfield, IA
Doug Wallace Ecologist ACES Program Columbia, MO
This site was originally published by Lisa Kluesner on 7/1/2019.
Rangeland health reference sheet
Interpreting Indicators of Rangeland Health is a qualitative assessment protocol used to determine ecosystem condition based on benchmark characteristics described in the Reference Sheet. A suite of 17 (or more) indicators are typically considered in an assessment. The ecological site(s) representative of an assessment location must be known prior to applying the protocol and must be verified based on soils and climate. Current plant community cannot be used to identify the ecological site.
Author(s)/participant(s) | |
---|---|
Contact for lead author | |
Date | 04/13/2025 |
Approved by | Suzanne Mayne-Kinney |
Approval date | |
Composition (Indicators 10 and 12) based on | Annual Production |
Indicators
-
Number and extent of rills:
-
Presence of water flow patterns:
-
Number and height of erosional pedestals or terracettes:
-
Bare ground from Ecological Site Description or other studies (rock, litter, lichen, moss, plant canopy are not bare ground):
-
Number of gullies and erosion associated with gullies:
-
Extent of wind scoured, blowouts and/or depositional areas:
-
Amount of litter movement (describe size and distance expected to travel):
-
Soil surface (top few mm) resistance to erosion (stability values are averages - most sites will show a range of values):
-
Soil surface structure and SOM content (include type of structure and A-horizon color and thickness):
-
Effect of community phase composition (relative proportion of different functional groups) and spatial distribution on infiltration and runoff:
-
Presence and thickness of compaction layer (usually none; describe soil profile features which may be mistaken for compaction on this site):
-
Functional/Structural Groups (list in order of descending dominance by above-ground annual-production or live foliar cover using symbols: >>, >, = to indicate much greater than, greater than, and equal to):
Dominant:
Sub-dominant:
Other:
Additional:
-
Amount of plant mortality and decadence (include which functional groups are expected to show mortality or decadence):
-
Average percent litter cover (%) and depth ( in):
-
Expected annual annual-production (this is TOTAL above-ground annual-production, not just forage annual-production):
-
Potential invasive (including noxious) species (native and non-native). List species which BOTH characterize degraded states and have the potential to become a dominant or co-dominant species on the ecological site if their future establishment and growth is not actively controlled by management interventions. Species that become dominant for only one to several years (e.g., short-term response to drought or wildfire) are not invasive plants. Note that unlike other indicators, we are describing what is NOT expected in the reference state for the ecological site:
-
Perennial plant reproductive capability:
Print Options
Sections
Font
Other
The Ecosystem Dynamics Interpretive Tool is an information system framework developed by the USDA-ARS Jornada Experimental Range, USDA Natural Resources Conservation Service, and New Mexico State University.
Click on box and path labels to scroll to the respective text.